10.3 Active Transport
Active transport mechanisms require the cell’s energy, usually in the form of adenosine triphosphate (ATP). If a substance must move into the cell against its concentration gradient—that is, if the substance’s concentration inside the cell is greater than its concentration in the extracellular fluid (and vice versa)—the cell must use energy to move the substance. Some active transport mechanisms move small-molecular weight materials, such as ions, through the membrane. Other mechanisms transport much larger molecules.
Moving Against a Gradient
To move substances against a gradient, the cell must use energy. This energy comes from the hydrolysis of ATP. Active transport mechanisms, or pumps, work against gradients. Small substances constantly pass through plasma membranes. Active transport maintains concentrations of ions and other substances that living cells require in the face of these passive movements. A cell may spend much of its metabolic energy supply maintaining these processes. (A red blood cell uses most of its metabolic energy to maintain the imbalance between exterior and interior sodium and potassium levels that the cell requires.) Because active transport mechanisms depend on a cell’s metabolism for energy, they are sensitive to many metabolic poisons that interfere with the ATP supply.
Two active transport mechanisms exist for transporting ions and small molecules. Primary active transport moves substances across a membrane against their gradients, using energy in the process. Secondary active transport does not directly require ATP: instead, it couples the active transport of a substance to the movement of an ion down its gradient.
Carrier Proteins for Active Transport
An important membrane adaptation for active transport is the presence of specific carrier proteins or pumps to facilitate movement: there are three varieties of transport proteins. A uniporter carries one specific ion or molecule. A symporter carries two different ions or molecules, both in the same direction. An antiporter also carries two different ions or molecules, but in different directions. All of these transporters can also transport small, uncharged organic molecules like glucose. These three types of carrier proteins are also used in facilitated diffusion, but they do not require ATP to work in that process. Some examples of pumps for active transport are Na+-K+ ATPase, which carries sodium and potassium ions, and H+-K+ ATPase, which carries hydrogen and potassium ions. Both of these are antiporter carrier proteins. Two other carrier proteins are Ca2+ ATPase and H+ ATPase, which carry only calcium and only hydrogen ions, respectively. Both are pumps.
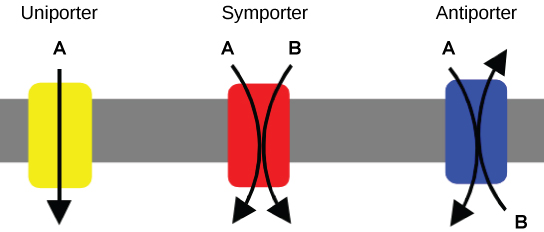
Primary Active Transport
Primary active transport is directly moving a substance across a membrane from an area of lower concentration to an area of higher concentration. Moving materials against a gradient requires cellular energy, which is generally supplied by ATP.
Let’s examine the sodium-potassium pump as an example of primary active transport.
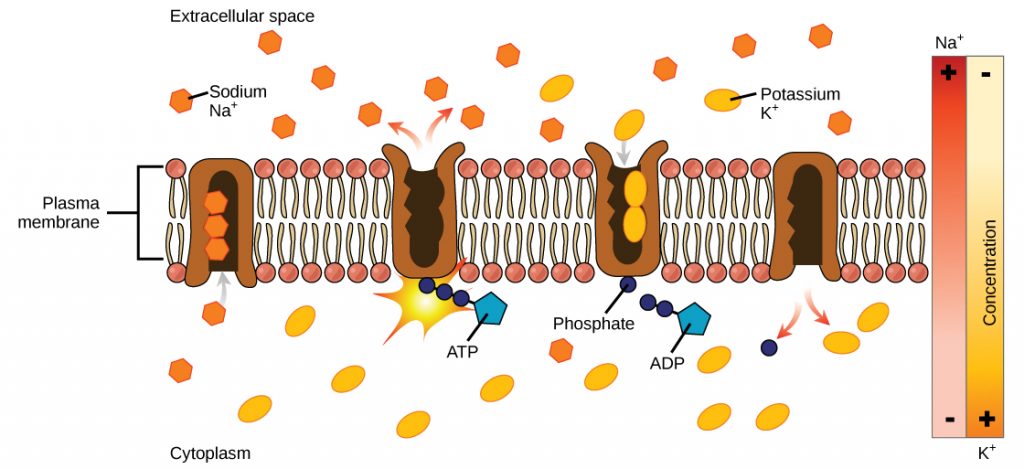
One of the most important pumps in animal cells is the sodium-potassium pump (Na+-K+ ATPase), which maintains the electrochemical gradient (and the correct concentrations of Na+ and K+) in living cells. The sodium-potassium pump moves K+ into the cell while moving Na+ out at the same time, at a ratio of three Na+ for every two K+ ions moved in. The Na+-K+ ATPase exists in two forms, depending on its orientation to the cell’s interior or exterior and its affinity for either sodium or potassium ions. The process consists of the following six steps.
- With the enzyme oriented towards the cell’s interior, the carrier has a high affinity for sodium ions. Three ions bind to the protein.
- The protein carrier hydrolyzes ATP and a low-energy phosphate group attaches to it.
- As a result, the carrier changes shape and reorients itself towards the membrane’s exterior. The protein’s affinity for sodium decreases and the three sodium ions leave the carrier.
- The shape change increases the carrier’s affinity for potassium ions, and two such ions attach to the protein. Subsequently, the low-energy phosphate group detaches from the carrier.
- With the phosphate group removed and potassium ions attached, the carrier protein repositions itself towards the cell’s interior.
- The carrier protein, in its new configuration, has a decreased affinity for potassium, and the two ions move into the cytoplasm. The protein now has a higher affinity for sodium ions, and the process starts again.
Secondary Active Transport (Co-transport)
Secondary active transport uses the kinetic energy of the diffusion of ions to bring other substances into the cell, against their concentration gradient . For example, sodium ion concentrations build outside of the plasma membrane because of the primary active transport process. If a channel protein exists and is open, the sodium ions will move down the concentration gradient across the membrane. This movement transports other substances that must be attached to the same transport protein in order for the sodium ions to move across the membrane. Many amino acids, as well as glucose, enter a cell this way.
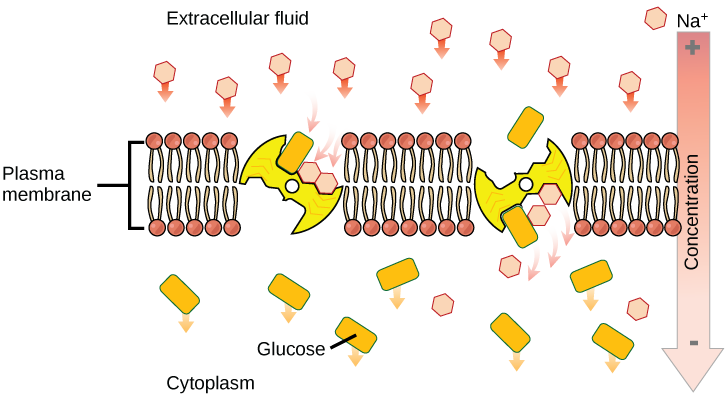
Another example is the storage of high-energy hydrogen ions in the mitochondria of plant and animal cells in order to produce ATP. The potential energy that accumulates in the hydrogen ion gradient translates into kinetic energy as the ions move through the channel protein ATP synthase, and that energy then converts ADP into ATP.
method of transporting material that requires energy
active transport mechanism that works against electrochemical gradients
active transport that moves ions or small molecules across a membrane and may create a difference in charge across that membrane
movement of material that results from primary active transport to the electrochemical gradient
transporter that carries one specific ion or molecule
transporter that carries two different ions or small molecules, both in the same direction
transporter that carries two ions or small molecules in different directions
process by which material moves down a concentration gradient (from high to low concentration) using integral membrane proteins