7.3 Protein Structure
Protein Structure
A protein’s shape is critical to its function. For example, an enzyme can bind to a specific substrate at an active site. If this active site is altered because of local changes or changes in overall protein structure, the enzyme may be unable to bind to the substrate. To understand how the protein gets its final shape or conformation, we need to understand the four levels of protein structure: primary, secondary, tertiary, and quaternary.
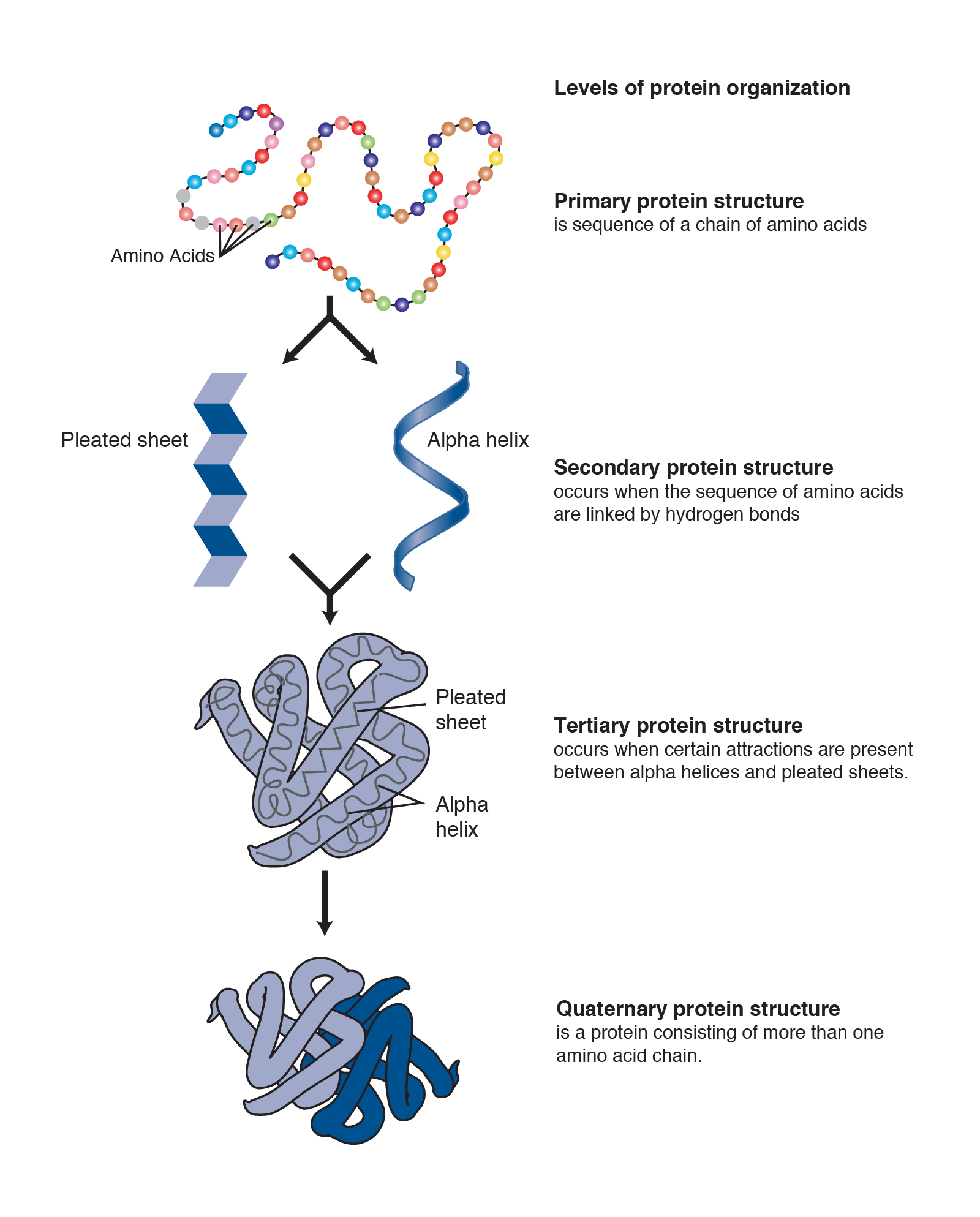
Primary Structure
The unique sequence of amino acids in a polypeptide chain is its primary structure. For example, the primary sequence of human cytochrome c protein is shown below.

The gene encoding the protein ultimately determines the unique sequence for every protein. A change in nucleotide sequence of the gene’s coding region may lead to adding a different amino acid to the growing polypeptide chain, causing a change in protein structure and function.
For example, in sickle cell anemia, the hemoglobin β-chain has a single amino acid substitution, causing a change in protein structure and function. Specifically, glutamic acid in the β-chain is replaced by valine. What is most remarkable to consider is that a hemoglobin molecule is comprised of two alpha and two beta chains that each consist of about 150 amino acids. The molecule, therefore, has about 600 amino acids. The structural difference between a normal hemoglobin molecule and a sickle cell molecule—which dramatically decreases life expectancy—is a single amino acid out of the 600.
Because of this change of one amino acid in the chain, hemoglobin molecules form long fibers that distort the biconcave, or disc-shaped, red blood cells and causes them to assume a crescent or “sickle” shape, which clogs blood vessels. This can lead to myriad serious health problems such as breathlessness, dizziness, headaches, and abdominal pain for those affected by this disease.
Secondary Structure
The local folding of the polypeptide in some regions gives rise to the secondary structure of the protein. The most common are the α-helix and β-pleated sheet (or simply β-sheet) structures. Both structures are held in shape by hydrogen bonds. The hydrogen bonds form between the oxygen atom in the carbonyl group in one amino acid and another amino acid that is four amino acids farther along the chain.
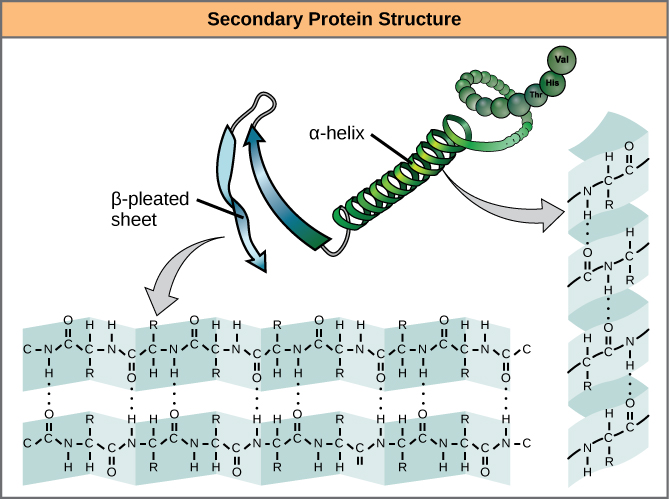
Every helical turn in an α–helix has 3.6 amino acid residues. The polypeptide’s R groups protrude out from the α-helix chain. In the β-pleated sheet, hydrogen bonding between atoms on the polypeptide chain’s backbone form the “pleats”. The R groups are attached to the carbons and extend above and below the pleat’s folds. The pleated segments align parallel or antiparallel to each other, and hydrogen bonds form between the partially positive hydrogen atom in the amino group and the partially negative oxygen atom in the peptide backbone’s carbonyl group.
Tertiary Structure
The polypeptide’s unique three-dimensional structure is its tertiary structure. This structure is in part due to chemical interactions. The interactions among R groups are most important for creating the protein’s complex three-dimensional tertiary structure. For example, R groups with like charges repel each other and those with unlike charges are attracted to each other (ionic bonds). When protein folding takes place, the nonpolar amino acids’ hydrophobic R groups tend to be in the protein’s interior; whereas, the hydrophilic R groups tend to be on the outside. Interaction between cysteine side chains forms disulfide bonds in the presence of oxygen, the only covalent bond that forms during protein folding.
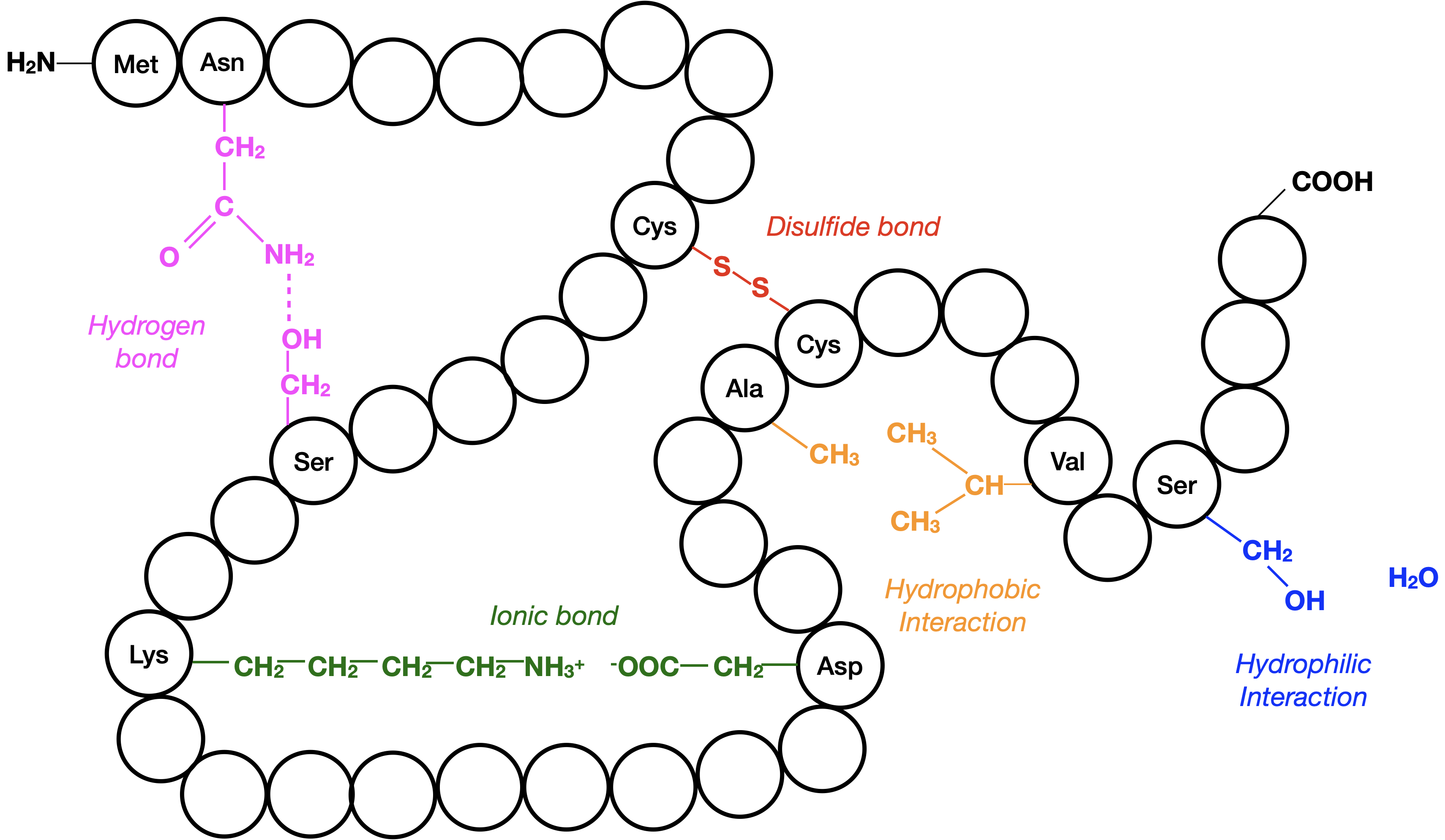
All of these interactions, weak and strong, determine the protein’s final three-dimensional shape. When a protein loses its three-dimensional shape, it may no longer be functional.
Quaternary Structure
In nature, some proteins form from several polypeptides, or subunits, and the interaction of these subunits forms the quaternary structure. Weak interactions between the subunits help to stabilize the overall structure. For example, insulin (a globular protein) has a combination of hydrogen and disulfide bonds that cause it to mostly clump into a ball shape. Insulin starts out as a single polypeptide and loses some internal sequences in the presence of post-translational modification after forming the disulfide linkages that hold the remaining chains together. Silk (a fibrous protein), however, has a β-pleated sheet structure that is the result of hydrogen bonding between different chains.
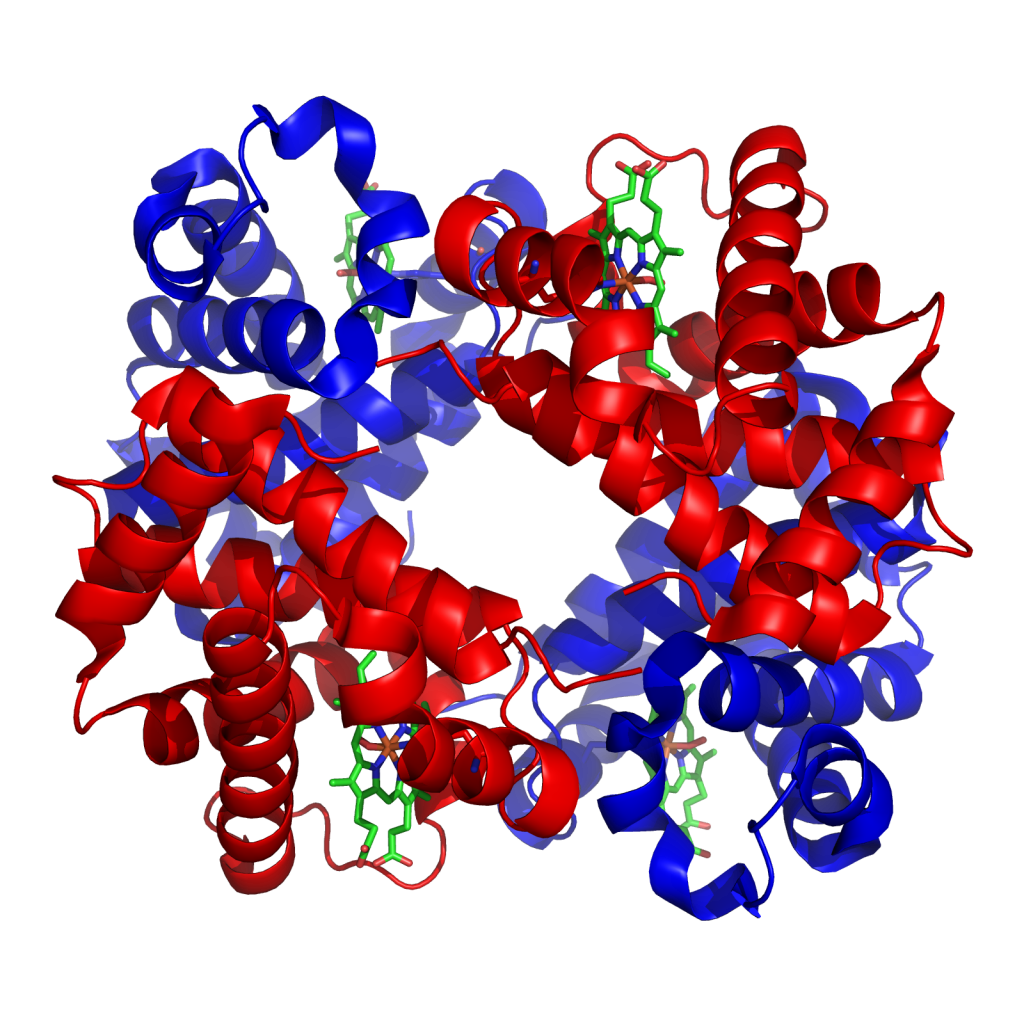
long chain of amino acids linked by peptide (covalent) bonds
linear sequence of amino acids in a protein
segment of DNA that codes for a functional molecule (usually, a protein)
regular structure that proteins form by intramolecular hydrogen bonding between the oxygen atom of one amino acid residue and the hydrogen attached to the nitrogen atom of another amino acid residue
type of secondary protein structure formed by folding the polypeptide into a helix shape with hydrogen bonds stabilizing the structure
secondary structure in proteins in which hydrogen bonding forms “pleats” between atoms on the polypeptide chain's backbone
a protein's three-dimensional conformation, including interactions between secondary structural elements; formed from interactions between amino acid side chains
covalent bond between two cysteines, formed by the joining of two sulfhydryl groups (R-S-S-R); important for protein structure
association of multiple polypeptide subunits in a protein