Oceans and Coastal Environments
7.2 Waves
Waves form on the ocean and lakes because energy from the wind is transferred to the water. Therefore, the stronger the wind, the longer it blows, and the larger the area of water over which it blows (the fetch), the larger the waves are likely to be.
The essential parameters of a wave are its wavelength (the horizontal distance between two crests or two troughs), its amplitude (the vertical distance between a trough and a crest), and its velocity (the speed at which wave crests move across the water). Relatively small waves move up to about 10 km/h and arrive on a shore about once every 3 seconds. Huge waves move about five times faster (over 50 km/h), but because their wavelengths are so much longer, they arrive less often – about once every 14 seconds. (17.1 Waves – Physical Geology, n.d.)
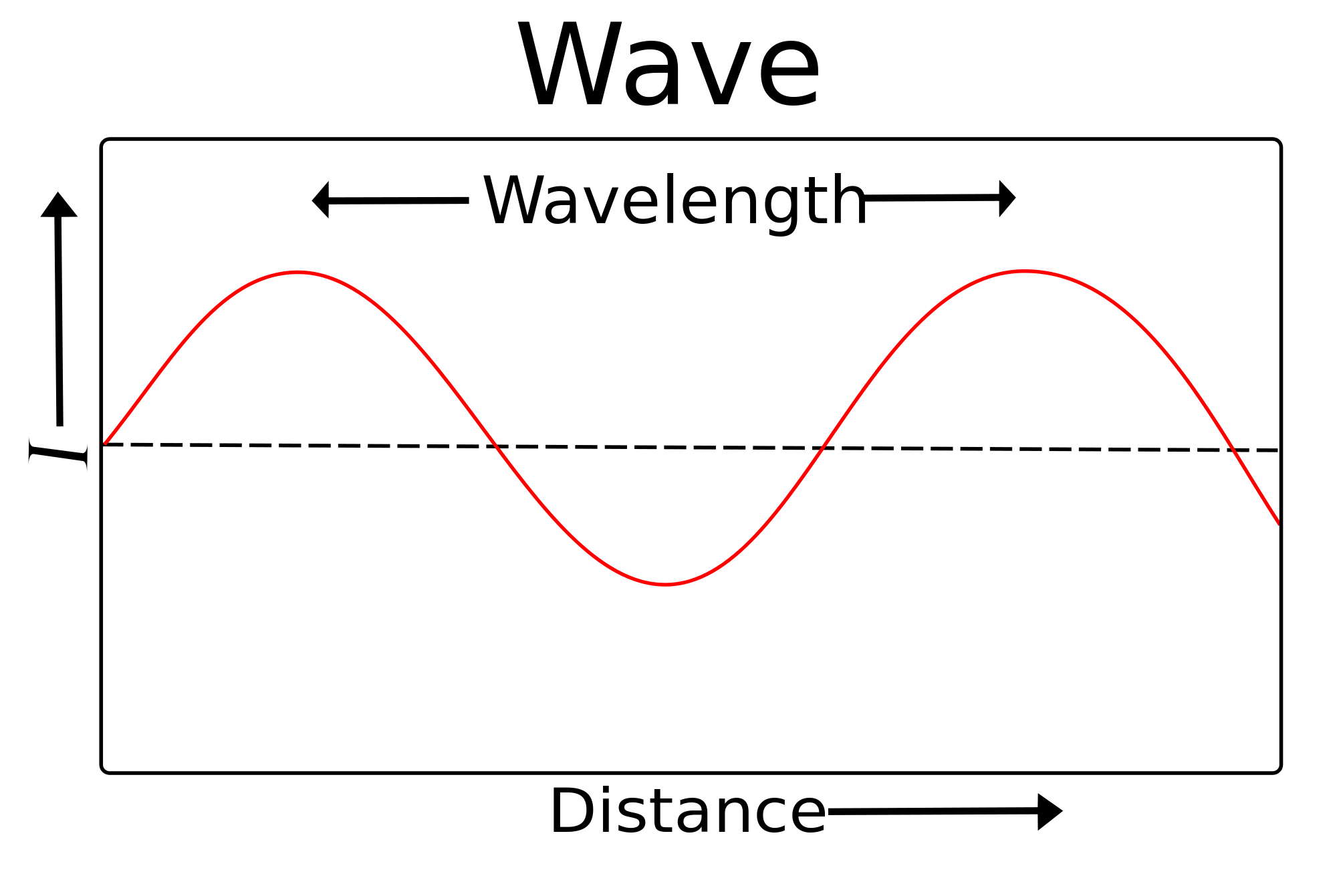
As a wave moves across the water’s surface, the water itself mostly moves up and down and only moves a small amount in the direction of wave motion. As this happens, a point on the water surface describes a circle with a diameter equal to the wave amplitude. This motion is also transmitted to the water underneath, and the water is disturbed by a wave to a depth of approximately one-half of the wavelength.
The one-half wavelength depth of disturbance of the water beneath a wave is known as the wave base. Since ocean waves rarely have wavelengths higher than 200 m, and the open ocean is several thousand meters deep, the wave base does not frequently interact with the ocean’s bottom. However, as waves approach the much shallower water near the shore, they start to “feel” the bottom, which is affected by that interaction. As a result, the wave “orbits” are both flattened and slowed by dragging, and the implications are that the wave amplitude (height) increases, and the wavelength decreases (the waves become much steeper). The ultimate result is that the waves lean forward and eventually break. (17.1 Waves – Physical Geology, n.d.)
Waves usually approach the shore at an angle, and this means that one part of the wave feels the bottom sooner than the rest of it, so the part that touches the bottom first slows down first. In open water, these waves had wavelengths close to 100 m. However, in the shallow water closer to shore, the wavelengths decreased to around 50 m, even less in some cases.
Even though they bend and become parallel to the shore, most waves still reach the shore at a slight angle, and as each one arrives, it pushes water along the shore, creating what is known as a longshore current within the surf zone where waves are breaking. (Ocean Movements | Earth Science, n.d.)
Another significant effect of waves reaching the shore at an angle is that when they wash up onto the beach, they do so at an angle, but when that same wave water washes back down the coast, it moves straight down the slope of the beach. The upward-moving water, known as the swash, pushes sediment particles along the beach, while the downward-moving water, the backwash, brings them straight back. With every wave that washes up and down the coast, sediment particles are moved along the beach in a zigzag pattern.
The combined effects of sediment transport within the surf zone by the longshore current and sediment movement along the beach by swash and backwash are known as longshore drift. Longshore drift moves a tremendous amount of sediment along coasts (both oceans and large lakes) around the world, and it is responsible for creating a variety of depositional features.
A rip current is another type of current that develops in the nearshore area and has the effect of returning water pushed up to the shore by incoming waves. Rip currents flow straight out from the shore and are fed by the longshore currents. They die out quickly outside the surf zone but can be dangerous to swimmers who get caught in them. If part of a beach does not have a strong unidirectional longshore current, the rip currents may be fed by longshore currents going in both directions.
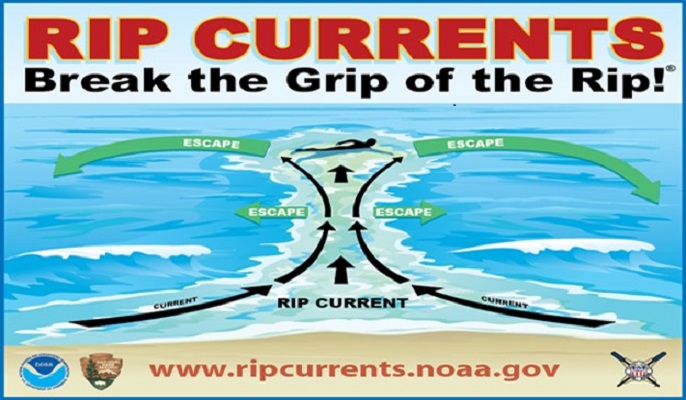
Behavior of Waves Approaching Shore
In the open sea, waves generally appear choppy because wave trains from many directions interact. Where crests converge with other crests, called constructive interference, they add together, producing peaks, a process referred to as wave amplification. Constructive interference of troughs produces hollows. Where crests converge with troughs, they cancel each other out, called destructive interference. As waves approach the shore and begin to make frictional contact with the seafloor (i.e., water depth is a half wavelength or less), they begin to slow down, but the energy carried by the wave remains the same, so they build up higher. The water moves in a circular motion as the wave passes, with the water that feeds each circle being drawn from the trough in front of the advancing wave. As the wave encounters shallower water at the shore, there is eventually insufficient water in front of the wave to supply a complete circle, and the crest pours over, creating a breaker. (12 Coastlines – An Introduction to Geology, n.d.)
Some of the damage done by storms is from storm surges. As storm winds push waves into the coast, the water piles up at a shoreline. Storm surge may raise sea level by 7.5 m (25 ft), devastating in a shallow land area when winds, waves, and rain are heavy.
Tsunamis
An energetic event affecting the seafloor generates a particular wave, such as earthquakes, submarine landslides, and volcanic eruptions. Called tsunamis, these waves are created when a portion of the seafloor is suddenly elevated by movement in the crustal rocks below that are involved in an earthquake. As a result, the water is suddenly lifted, and a wave train spreads out in all directions from the mound carrying enormous energy and traveling very fast (hundreds of miles per hour).
Tsunamis may pass unnoticed in the open ocean because the wavelength is very long and shallow wave height. However, as the wave train approaches the shore, each wave makes contact with the shallow seafloor, friction increases, and the wave slows down. Wave height builds up, and the wave strikes the shore as a wall of water a hundred or more feet high. The massive wave may sweep inland well beyond the beach. This is called the tsunami run-up, which destroys structures far inland. Tsunamis deliver a catastrophic blow to observers at the beach as the water in the trough in front of it is drawn back toward the tsunami wave, exposing the seafloor. Curious and unsuspecting people on the beach may run out to see exposed offshore sea life only to be overwhelmed when the breaking crest hits. (12 Coastlines – An Introduction to Geology, n.d.)
Tidal Waves
Tides are the daily rise and fall of sea level at any given place. The pull of the Moon’s gravity on Earth is the primary cause of tides, and the pull of the Sun’s gravity on Earth is the secondary cause. The Moon has a more significant effect because, although it is much smaller than the Sun, it is much closer. The Moon’s pull is about twice that of the Sun’s.
Daily Tide Patterns
To understand the tides, it is easiest to start with the effect of the Moon on Earth. As the Moon revolves around our planet, its gravity pulls Earth toward it. The lithosphere cannot move much, but gravity pulls the water above it, creating a bulge. This bulge is the high tide beneath the Moon. The Moon’s gravity then pulls the Earth toward it, leaving the water on the opposite side of the planet behind. This creates a second-high tide bulge on the opposite side of Earth from the Moon. The high tides of these two water bulges on opposite sides of the Earth aligned with the Moon. (Tides | Earth Science, n.d.)
Since so much water is pulled into the two high tides, low tides form between the two high tides. As the Earth rotates beneath the Moon, a single spot will experience two high tides and two low tides every day.
The tidal range is the difference between the ocean level at high tide and the ocean at low tide. The tidal range in a location depends on some factors, including the seafloor slope. Water appears to move a greater distance on a gentle slope than on a steep slope. (Ocean Movements | Earth Science, n.d.)
Monthly Tidal Patterns
Waves are additive, so when the gravitational pull of the Sun and Moon are in the same direction, the high tides add, and the low tides add. As a result, high tides and low tides are lower than at other times throughout the month. With a greater tidal range, these more extreme tides are called spring tides. Spring tides do not just occur in the spring; they occur whenever the Moon is in a new-moon or full-moon phase every 14 days. (Tidal Waves | Physical Geography, n.d.)
Neap tides have the smallest tidal range, and they occur when the Earth, the Moon, and the Sun form a 90-degree angle. They occur precisely halfway between the spring tides when the Moon is first or last quarter. How do the tides add up to create neap tides? The Moon’s high tide occurs in the same place as the Sun’s low tide and the Moon’s low tide in the same place as the Sun’s high tide. At neap tides, the tidal range is relatively small.
High tides occur about twice a day, every 12 hours and 25 minutes. The reason is that the Moon takes 24 hours and 50 minutes to rotate once around the Earth, so the Moon is over the exact location 24 hours and 50 minutes later. Therefore, since high tides occur twice a day, one arrives every 12 hours and 25 minutes. (Tides | Earth Science, n.d.)
Some coastal areas do not follow this pattern at all. These coastal areas may have one high and one low tide per day or a different time between two high tides. These differences are often because of local conditions, such as the shape of the coastline that the tide is entering. The National Ocean Service has a wealth of information on tides and water levels.