Tectonic Forces
4.5 Volcanism
Magma Generation
Magma and lava contain melt, solids, and volatiles (dissolved gases). The liquid part, called melt, is made of ions from minerals that have already melted. The solid piece, called solids, are crystals of minerals that have not melted (higher melting temperature) and are floating in the melt. Finally, volatiles is gaseous components dissolved in magma, such as water vapor, carbon dioxide, sulfur, and chlorine. The presence and amount of these three components affect the physical behavior of magma. (4 Igneous Processes and Volcanoes – An Introduction to Geology, n.d.)
Geothermal Gradient
Although it is scorching under the Earth’s surface, the crust and mantle are mostly solid. This heat inside the Earth is caused by residual heat leftover from the original formation of Earth and radioactive decay. The rate at which temperature increases with depth is called the geothermal gradient. The average geothermal gradient in the upper 100 kilometers of the crust is generally about 25 degrees Celsius per kilometer (km). So, for every kilometer of depth, the temperature increases by about 25 degrees Celsius. (4 Igneous Processes and Volcanoes – An Introduction to Geology, n.d.)

Decompression Melting
Magma is created at the mid-ocean ridge by decompression melting. The mantle is solid but is slowly flowing under enormous pressure and temperatures due to convection. Rock is not a good conductor of heat, so as mantle rock rises, the pressure is reduced along with the melting point (the green line), but the rock temperature remains about the same, and the rising rock begins to melt. Pressure changes instantaneously as the rock rises, but temperature changes slowly because of the low heat conductivity of the rock. In the figure above, setting B: mid-ocean ridge shows a mass of mantle rock at a pressure-temperature X on the P-T diagram and its geographical location on the cross-section under a mid-ocean ridge. The P-T diagram shows the red arrow increasing to the right at this location. Thus, hotter rock is now shallower, at a lower pressure, and the new geothermal gradient (red line) shifts past the solidus (green line), and melting starts. As this magma continues to rise at divergent boundaries and encounters seawater, it cools and crystallizes to form new lithospheric crust. (4 Igneous Processes and Volcanoes – An Introduction to Geology, n.d.)
Flux Melting
Another way that rocks melt is when volatile gases (e.g., water vapor) are added to mantle rock from a descending subducting slab in a process called flux melting (or fluid-induced melting). The subducting slab contains oceanic lithosphere and hydrated minerals. As the slab descends and slowly increases in temperature, volatiles is expelled from these hydrated minerals, like squeezing water out of a sponge. The volatiles then rises into the overlying asthenospheric mantle, which lowers the melting point of the peridotite minerals (olivine and pyroxene). The pressure and temperature of the overlying mantle rock do not change, but the addition of volatiles lowers the melting temperature. This is analogous to adding salt to an icy roadway. The salt lowers the melting/crystallization temperature of the solid water (ice) to melt. Another example is welders adding flux to reduce the melting point of their welding materials.
Flux melting is illustrated in setting D: island arc (subduction zone) of the P-T diagram above. Volatiles added to mantle rock at location “Z” act as a flux to lower the melting temperature. This is shown in the P-T diagram by the solidus (green line) shifting to the left. The solidus line moves past the geothermal gradient (red line), melting begins. Magmas producing the volcanoes of the Ring of Fire, associated with the circum-Pacific subduction zones, are a result of flux melting. As introduced in the minerals chapter, water ions can bond with other ions in the crystal structures of amphibole (and other silicates), which is essential in considering how magmas form in subduction zones by “flux melting.” Such hydrated minerals in subducting slabs contribute water to the flux melting process. (4 Igneous Processes and Volcanoes – An Introduction to Geology, n.d.)
Magma Composition
In 1980, Mount St. Helens blew up in United States history’s costliest and deadliest volcanic eruption. The eruption killed 57 people, destroyed 250 homes, and swept away 47 bridges. Mount St. Helens today still has minor earthquakes and eruptions and now has a horseshoe-shaped crater with a lava dome inside. The dome is formed of viscous lava that oozes into place.
It should first be noted that magma is molten material inside the Earth, while lava is molten on its surface. The distinction is because lava can cool quickly from the air and solidify into rock rapidly, while magma may never reach the Earth’s surface. As a result, volcanoes do not always erupt in the same way. Each volcanic eruption is unique, differing in size, style, and composition of the erupted material. One key to what makes the eruption unique is the chemical composition of the magma that feeds a volcano, which determines (1) the eruption style, (2) the type of volcanic cone that forms, and (3) the composition of rocks that are found at the volcano.
Different minerals within rocks melt at different temperatures, and the amount of partial melting and the composition of the original rock determine the magma’s composition. Magma collects in magma chambers in the crust at 160 kilometers (100 miles) beneath the surface of a volcano.
The words that describe the composition of igneous rocks also describe magma composition. Mafic magmas are low in silica and have darker magnesium and iron-rich mafic minerals, such as olivine and pyroxene. Felsic magmas are higher in silica and have lighter colored minerals such as quartz and orthoclase feldspar. The higher the amount of silica in the magma, the higher is its viscosity. Viscosity is a liquid’s resistance to flow.
Viscosity determines what magma will do. Mafic magma is not viscous and will flow smoothly to the surface. Felsic magma is viscous and does not flow smoothly. Most felsic magma will stay deeper in the crust and cool to form intrusive igneous rocks such as granite and granodiorite. If felsic magma rises into a magma chamber, it may be too viscous to move, so it gets stuck. Dissolved gases become trapped by thick magma, and the magma chamber begins to build pressure.
Explosive Eruptions
The type of magma in the chamber determines the type of volcanic eruption. A massive explosive eruption creates even more devastation than the force of the atom bomb dropped on Nagasaki at the end of World War II, in which more than 40,000 people died. A massive explosive volcanic eruption is 10,000 times as powerful. Felsic magmas erupt explosively because of hot, gas-rich magma churning within its chamber. The pressure becomes so great that the magma eventually breaks the seal and explodes, like when a cork is released from a champagne bottle. Magma, rock, and ash burst upward in an enormous explosion, creating tephra volcanic ash. When looked under a microscope, the volcanic “ash” is actual microscopic shards of glass when examined under a microscope. That is why it is so dangerous to inhale the air following an eruption.

As a pyroclastic flow, scorching hot tephra, ash, and gas may speed down the volcano’s slopes at 700 km/h (450 mph). Pyroclastic flows knock down everything in their path. The temperature inside a pyroclastic flow may be as high as 1,000 degrees Celsius (1,800 degrees Fahrenheit). Before the Mount St. Helens eruption in 1980, the Lassen Peak eruption on May 22, 1915, was the most recent Cascade eruption. A column of ash and gas shot 30,000 feet into the air. This triggered a high-speed pyroclastic flow, which melted snow and created a volcanic mudflow known as a lahar. Lassen Peak currently has geothermal activity and could erupt explosively again. Mt. Shasta, the other active volcano in California, erupts every 600 to 800 years. An eruption would create a large pyroclastic flow and probably a lahar. Then, of course, Mt. Shasta could explode and collapse like Mt. Mazama in Oregon.
Volcanic gases can form toxic and invisible clouds in the atmosphere, contributing to environmental problems such as acid rain and ozone destruction. In addition, dust and ash particles may stay in the atmosphere for years, disrupting weather patterns and blocking sunlight.
Effusive Eruptions
Mafic magma creates gentler effusive eruptions. Although the pressure builds enough for the magma to erupt, it does not erupt with the same explosive force as felsic magma. As a result, people can usually be evacuated before an effusive eruption, much less deadly. Magma pushes toward the surface through fissures and reaches the surface through volcanic vents. Click here to view a lava stream within the vent of a Hawaiian volcano using a thermal camera.
Low-viscosity lava flows down mountainsides. Differences in composition and where the lavas erupt result in lava types like a ropy form pahoehoe and a chunky form called aa. Although effusive eruptions rarely kill anyone, they can be destructive. Even when people know that a lava flow is approaching, there is not much anyone can do to stop it from destroying a building, road, or infrastructure.

Volcano Features and Types
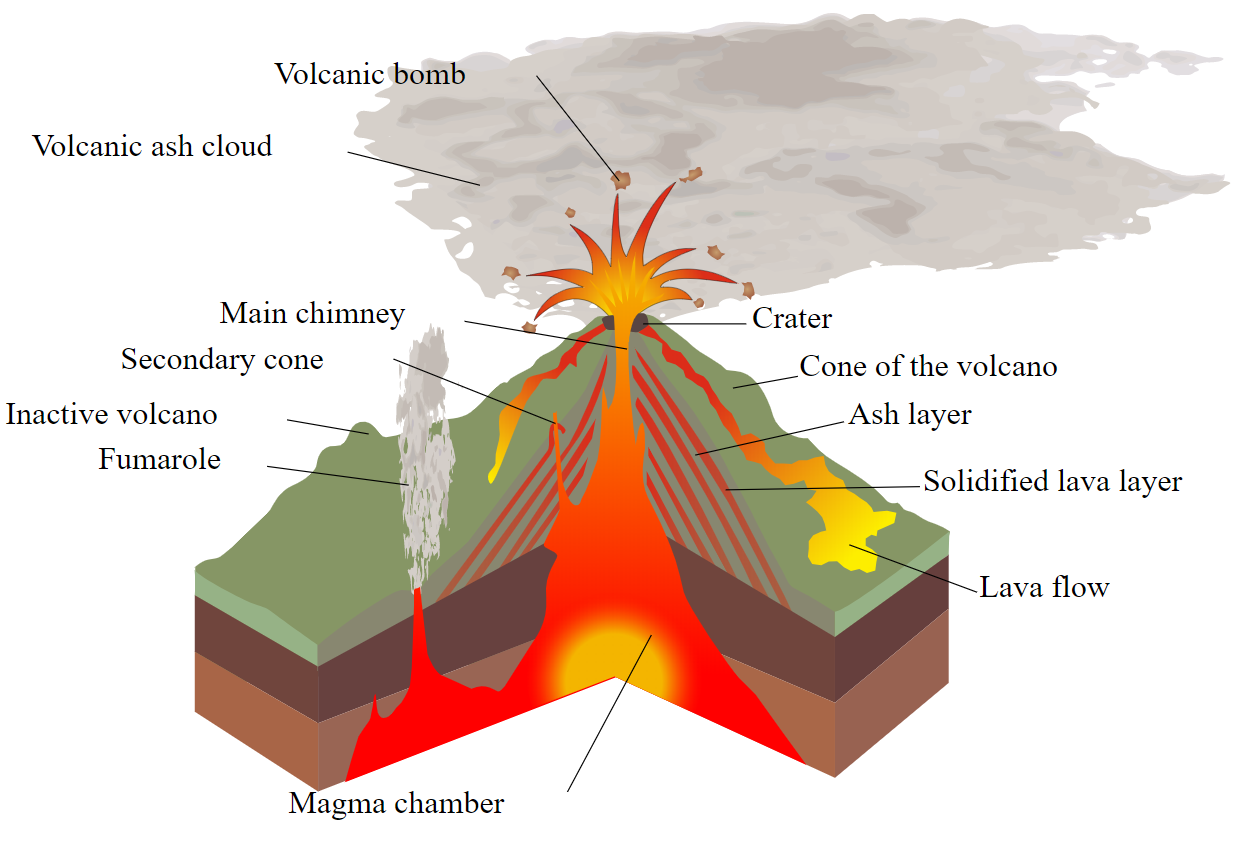
A volcano occurs where lava erupts at the surface and solidifies into rock. Several volcanoes are based on their shape, eruption style, magmatic composition, and other aspects. The most massive craters are called a caldera, such as the Crater Lake Caldera in Oregon. Many volcanic features are produced by viscosity, a fundamental property of lava. Viscosity is the resistance to flowing by a fluid. Low viscosity magma flows easily, more like syrup, the basaltic volcanism in Hawaii on shield volcanoes. High viscosity means a sticky magma, typically felsic or intermediate, that flows slowly, like toothpaste. (4 Igneous Processes and Volcanoes – An Introduction to Geology, n.d.)
Shield Volcanoes
The largest volcano is a shield volcano characterized by broad, low-angle flanks, a small vent, or groups of vents at the top, and basaltic magma. The name “shield” comes from the side view resembling a medieval warrior’s shield. They are typically associated with hotspots, midocean ridges, or continental rifts where upper mantle material rises and builds up slowly from much low-viscosity basaltic lava flows that can travel long distances, making the low-angle flanks. Because the magma is basaltic and has low viscosity, the eruption style is not explosive but rather effusive, meaning that volcanic eruptions are small, localized, and predictable. Therefore, this eruption style is not typically much of a hazard.
Mauna Loa and the more active Kilauea in Hawaii are good examples of vents on a shield volcano. The eruption of Kilauea from fissures in Hawaii in 2018, while not explosive, produced viscous lavas that did considerable damage to roads and structures. Shield volcanoes are also found in Iceland, the Galapagos Islands, Northern California, Oregon, and the East African Rift (USGS, 2011).

Basaltic magma can form several rock types and unique landforms. Based on magma temperature, composition, and content of dissolved gases and water vapor, there are two main types of basaltic volcanic rocks with Hawaiian names – pahoehoe and aa. Pahoehoe is a basaltic magma that flows smoothly into a “ropey” appearance. In contrast, aa (sometimes spelled a’a or ʻaʻā and pronounced “ah-ah”) has a crumbly blocky appearance. (Peterson and Tilling 1980). Felsic silica-rich lavas also form aa flows.
In basaltic lava flows, the low viscosity lava can smoothly flow, and it tends to harden on the outside but continue to flow internally within a tube. Once the interior flowing lava subsides, the tube may be left as an empty lava tube. Lava tubes famously make caves (with or without collapsed roofs) in Hawaii, Northern California, the Columbia River Basalt Plateau of Washington and Oregon, El Malpais National Monument in New Mexico, and Craters of the Moon National Monument in Idaho. Fissures, cracks that originate from shield-style eruptions are also common. Magmas from fissures are typically very fluid and mafic. The volcanic activity itself causes some fissures, and some can be influenced by tectonics, such as the common fissures parallel to the divergent boundary in Iceland. See above for fissure flows from Kilauea in 2018.
Since basalt flows are thick accumulations of lava with a homogeneous composition that flows quickly when the lava begins to cool, it can contract into columns with a hexagonal cross-section called columnar jointing. This feature is common in basaltic lava flows but can be found in more felsic lavas and tuffs. (4 Igneous Processes and Volcanoes – An Introduction to Geology, n.d.)
Cinder Cones
Cinder cones are small volcanoes with steep sides of cinders and volcanic bombs ejected from a clear central vent. Typically, they come from mafic lavas that have high volatile content. Cinders form when hot lava is ejected into the air, cooling and solidifying before reaching the volcano’s flank. The largest cinders are called volcanic bombs. Cinder cones form in short-lived eruption events common in the western United States.
A recent and striking example of a short-lived cinder cone is the 1943 eruption near Parícutin, Mexico. The cinder cone started with an explosive eruption shooting cinders out of a vent in the middle of a farmer’s field. Quickly, volcanism continued building the cone to a height of over 300 feet in a week and 1,200 feet in the first eight months. After the initial explosive gases and cinders were released, growing the cone, basaltic lava poured out around the base of the cone. This order of events is typical for cinder cones: first violent eruption, then the formation of cone and crater, followed by a low-viscosity lava flow from the base (the cone of cinders is not strong enough to support a column of lava rising to the top of the crater). The Parícutin cinder cone was built over nine years, covered about 100-square miles with ashes, and destroyed the town of San Juan.
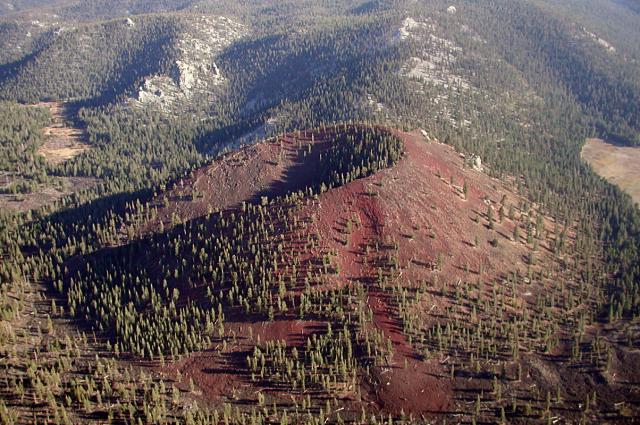
Lava Domes
Lava domes are a relatively small accumulation of silica-rich volcanic rocks, such as rhyolite and obsidian, that are too viscous to flow, and therefore, pile high close to the vent. The domes often form within the collapsed crater of a stratovolcano near the vent and grow by expansion from within. As it expands, its outer surface cools and hardens, then shatters, spilling loose fragments down its sides. An excellent example of a lava dome is inside of a collapsed stratovolcano crater is Mount Saint Helens. Examples of a stand-alone lava dome are Chaiten in Chile and the Mammoth Mountain in California. (Volcanoes: Principal Types of Volcanoes, n.d.)

Composite Volcanoes
Composite volcanoes, also called stratovolcanos, has steep flanks, a symmetrical cone shape, a distinct crater, and rise prominently above the surrounding landscape. The figure at the beginning of this section shows a stratovolcano. Examples include Mount Rainier in the Cascade Range in Washington and Mount Fuji in Japan. Stratovolcanoes can have magma with felsic to mafic composition. However, felsic to intermediate magmas are most common. “composite” refers to the alternating layers of pyroclastic materials (ash) and lava flows. The viscous nature of the intermediate and felsic magmas in subduction zones results in steep flanks and explosive eruption styles. Stratovolcanoes are made of alternating lava flows and ash. (4 Igneous Processes and Volcanoes – An Introduction to Geology, n.d.)
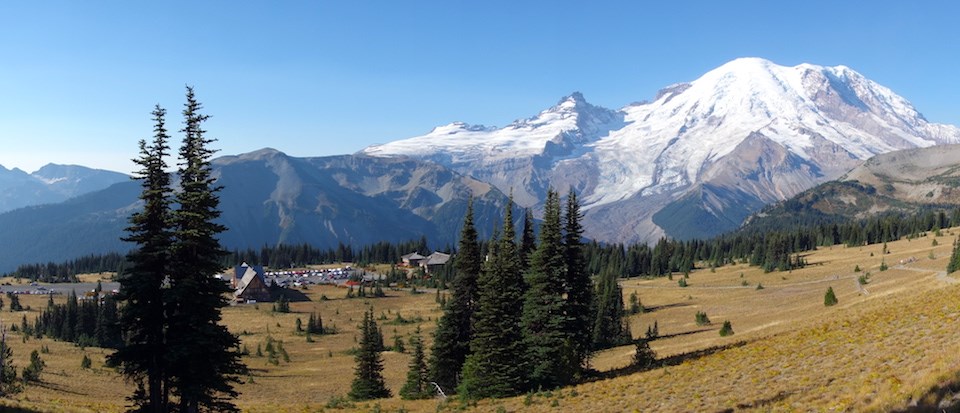
https://youtu.be/Qc0w9bklDB0
Calderas
Calderas are generally large (some up to 15 miles in diameter), steep-walled, basin-shaped depressions formed by the collapse of a volcanic edifice into an emptying magma chamber, and although the word caldera only refers to the vent, many use calderas as a volcano type, typically formed by high-viscosity felsic volcanism with high volatile content. Crater Lake, Yellowstone, and Long Valley Caldera are good examples. At Crater Lake National Park in Oregon, about 6,800 years ago, Mount Mazama was a composite volcano that erupted in a sizeable explosive blast, ejecting massive volcanic ash. The eruption rapidly drained the underlying magma chamber, causing the top to collapse, forming a significant depression later filled with water. Today a resurgent dome is found rising through the lake as a cinder cone, called Wizard Island.

Flood Basalts
A rare volcanic eruption type, unobserved in modern times, is the flood basalt. Flood basalts are some of the largest and lowest viscosity types of eruptions known. However, they are unknown from any eruption in human history, so the exact mechanisms of eruption are still debatable. Some famous examples include the Columbia River Flood Basalts in Washington, Oregon, and Idaho, the Deccan Traps, which cover about 1/3 of the country of India, and the Siberian Traps, which may have been involved in the Earth’s most massive mass extinction at the end of the Permian. (4 Igneous Processes and Volcanoes – An Introduction to Geology, n.d.)
Supervolcanoes
The Yellowstone caldera erupted three times recently, at 2.1, 1.3, and 0.64 million years ago. Each eruption created large rhyolite flows and pyroclastic clouds of ash that solidified into tuff. These extra-large eruptions rapidly emptied the magma chamber causing the roof to collapse and form a caldera. Three calderas are still preserved from these eruptions, and most of the roads and hotels of Yellowstone National Park are located within the caldera. Two resurgent domes are located within the last caldera.
Yellowstone volcanism started as a hot spot under the North American lithosphere about 17-million years ago near the Oregon/Nevada border. As the North American plate slid southwestward over the stationary hotspot, surface volcanism followed and helped form Idaho’s Snake River Plain, eventually arriving at its current location in northwestern Wyoming. As the plate moved to the southwest over the stationary hotspot, it left a track of past volcanic activities.

The Long Valley Caldera near Mammoth, California, is a massive explosive volcano that erupted 760,000 years ago and dumped a significant amount of ash throughout the United States, similar to the Yellowstone eruptions. This ash formed the large Bishop Tuff deposit. Like the Yellowstone caldera, the Long Valley Caldera contains the town of Mammoth Lakes, a major ski resort, an airport, and a major highway. Further, there is a resurgent dome in the middle and active hot springs. (4 Igneous Processes and Volcanoes – An Introduction to Geology, n.d.)
Distribution of Volcanic Activity
Most volcanoes are located at active plate boundaries called interplate volcanism. The prefix “inter-” means “between.” In contrast, some volcanoes are not associated with plate boundaries but are found within the plate far from plate boundaries. These are called intraplate volcanoes, and hotspots and fissure eruptions form many. The prefix “intra-” means “within.” The following discusses volcanism’s location in more detail with mid-ocean ridges, subduction zones, continental rifts representing interplate volcanism, and hot spots representing intraplate volcanism.
Volcanoes along Mid-Oceanic Ridges
Although most volcanism occurs on the ocean floor along the mid-ocean ridge (a type of divergent plate boundary), they are the least observed since most are under 10,000 to 15,000 feet of ocean, an exception being Iceland. As the oceanic plates diverge and thin, hot mantle rock is allowed to rise, pressure from depth is released, which causes the ultramafic mantle rock (peridotite) to melt partially. The resulting magma is basaltic in composition based on the concept of partial melting discussed earlier. Because most volcanoes on the ocean floor are basaltic, most of the oceanic lithosphere is also basaltic near the surface, with phaneritic gabbro and ultramafic peridotite forming underneath. Icelandic volcanism is an example of this, but lying above sea level.
An underwater volcanic eruption occurs when basaltic magma erupts underwater, forming pillow basalts or small explosive eruptions. Lava erupting into seawater forms pillow-shaped structures, hence the name. In association with these seafloor eruptions, an entire underwater ecosystem thrives in parts of the mid-ocean ridge. This ecosystem exists around tall vents emitting black, hot mineral-rich water called deep-sea hydrothermal vents (also known as black smokers).
Up to 380 degrees Celsius (716 degrees Fahrenheit), this hot water is heated by magma and dissolves many elements that support the ecosystem. Deep underwater where the sun cannot reach, this ecosystem of organisms depends on the vent’s heat for energy and vent chemicals as its foundation of life called chemosynthesis. The foundation of the ecosystem is hydrogen sulfide-oxidizing bacteria that live symbiotically with the larger organisms. Hydrogen sulfide (H2S, the gas that smells like rotten eggs) needed by these bacteria is contained in the volcanic gases emitted from the hydrothermal vents. The source of most of this sulfur and other elements is the Earth’s interior. (4 Igneous Processes and Volcanoes – An Introduction to Geology, n.d.)

Volcanoes along Convergent Boundaries
Volcanoes are a vibrant manifestation of plate tectonics processes. Volcanoes are common along convergent and divergent plate boundaries but are also found within lithospheric plates away from plate boundaries. Wherever mantle can melt, volcanoes may be the result. Volcanoes erupt because mantle rock melts. The first stage in creating a volcano is when mantle rock begins to melt because of scorching temperatures, lithospheric pressure lowers, or water is added.
Subduction Zones
Water is expelled from the hydrated minerals during subduction, causing partial melting by flux melting in the overlying mantle rock. This creates a mafic magma that rises through the lithosphere and can change the composition by interacting with the surrounding continental crust and magma differentiation. These changes evolve basaltic magma into a more silica-rich rock in volcanoes and plutons. These silica-rich rocks are felsic to intermediate rocks such as andesite, rhyolite, pumice, and tuff. The Ring of Fire surrounding the Pacific Ocean is dominated by subduction and contains volcanoes with silica-rich magma. These volcanoes are discussed in more detail in the stratovolcano section.
Large earthquakes are prevalent along convergent plate boundaries. Since the Pacific Ocean is rimmed by convergent and transform boundaries, 80 percent of all earthquakes occur around the Pacific Ocean basin. A description of the Pacific Ring of Fire along western North America is below:
- Subduction at the Middle American Trench creates volcanoes in Central America.
- The San Andreas Fault is a transform boundary.
- Subduction of the Juan de Fuca plate beneath the North American plate creates the Cascade volcanoes like Mount St. Helens, Mount Rainer, Mount Hood, and more.
- Subduction of the Pacific plate beneath the North American plate in the north creates the long chain of the Aleutian Islands volcanoes near Alaska.
Continental Rifts
In addition to volcanoes at the mid-ocean ridge and subduction zones, some volcanoes are at continental rifts, where the lithosphere is diverging and thinning, such as in the Basin and Range Province in North America and the East African Rift Basin in Africa. The thinning allows some of the lower crustal rocks or upper mantle rocks to rise, releasing some pressure and causing partial melting. The magma generated is less dense than the surrounding rock and rises through the crust to the surface, erupting as basalt. These basaltic eruptions are usually flood basalts, cinder cones, and basaltic lava flows. For example, young cinder cones are found in south-central Utah, the Black Rock Desert Volcanic Field, part of the Basin, and Range crustal extension. The 1-minute video (below) illustrates volcanism in the Basin and Range Province. These Utah cinder cones and lava flows started erupting 6 million years ago, with the last volcanic eruption 720 years ago. (4 Igneous Processes and Volcanoes – An Introduction to Geology, n.d.)
Hotspots
The primary source of intraplate volcanism is hotspots. Hotspots occur when lithospheric plates glide over a hot mantle plume, an ascending column of hot rock (solid, not magma) originating from deep within the mantle. A chain of ancient volcanoes formerly active but now inactive for millions of years can be seen on the seafloor or continents, leading to an active intraplate volcano, indicating hotspot volcanism. The Pacific oceanic plate overrode a hotspot mantle plume producing a long volcanic island chain beginning with the Emperor Seamounts in the northwest Pacific and terminating at the Hawaiian Islands with currently active volcanoes. When the North American continental plate overrode a mantle plume hotspot, a chain of ancient volcanic calderas formed, extending from Southwestern Idaho to the Yellowstone caldera. (4 Igneous Processes and Volcanoes – An Introduction to Geology, n.d.)
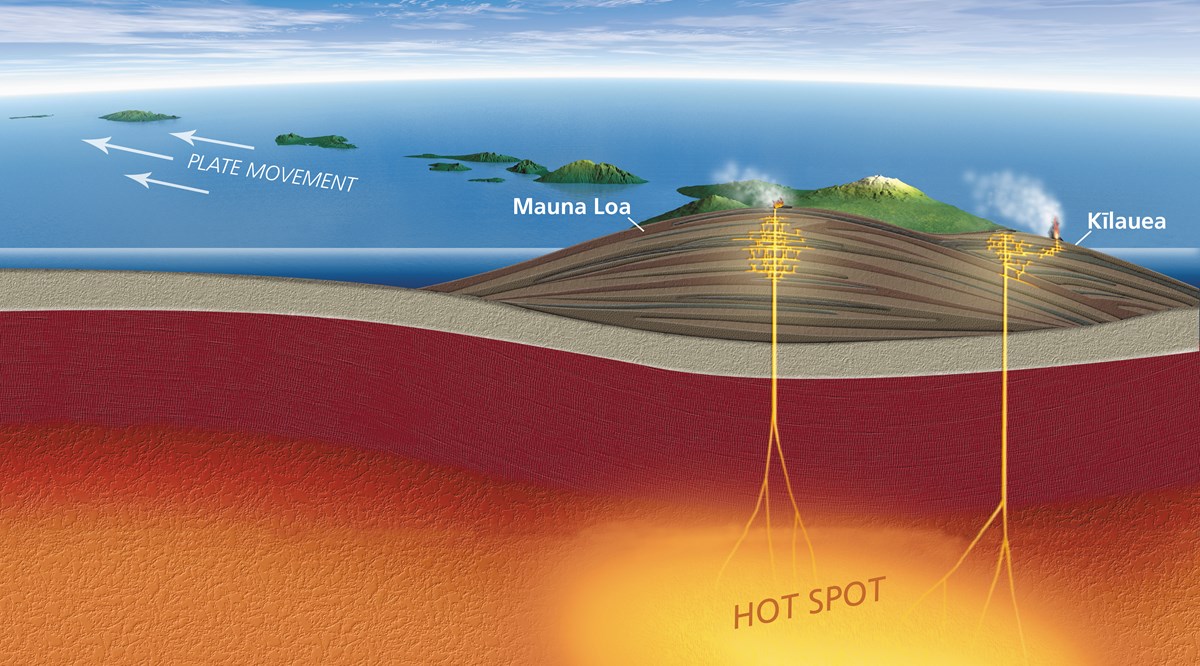
Once the ascending magma reaches the lithosphere, it spreads outward, tens to hundreds of kilometers across. Think of Bowen’s Reaction Series and the temperatures of the magmas that contain the respective minerals. If hot mafic magma rises beneath felsic continental crust spreads into a head below the felsic boundary, the higher heat of the mafic magma may cause the felsic rock above it to melt. There may be mixing the mafic material from below with the felsic above to form intermediate magmas. The felsic magma may melt and rise higher, creating granitic batholiths or even emerging as a felsic volcano. Such felsic (granitic) batholiths lie at the core of the Sierra Nevada Mountains and comprise Yosemite’s dramatic features. Since most mantle plumes are beneath the oceanic lithosphere, volcanism’s initial stages typically take place on the seafloor. Over time, basaltic volcanoes may form islands like those in Hawaii. Suppose the hotspot is under the continental lithosphere. In that case, the magma of more felsic to intermediate (silica-rich) composition rises into an explosive volcano-like Mt. St. Helens or the Yellowstone caldera.