Introduction to Geographic Science
1.4 Mapping Technology
Geographic Grid Systems
The Earth has 360 degrees and is measured using a grid pattern called the graticule. Lines of latitude and longitude allow any absolute location on the planet to have an identifiable address of degrees north or south and east or west, enabling geographers to locate, measure, and study spatial activity accurately.
Geographers and cartographers organize locations on the Earth using a series of imaginary lines that encircle the globe. The two primary lines are the equator and the prime meridian. The systems of longitude and latitude are formed from these lines, allowing users to locate themselves anywhere on the planet. The line is the longest when one travels along in an east-west direction. At the equator, the sun is directly overhead at noon on the two equinoxes, which occur in March and September.
Latitude and Parallels
Latitude is the angular distance of a place north or south of the Earth’s equator, usually expressed in degrees and minutes or decimal degrees. Lines connecting points of latitude are called parallels. The equator is the largest circle of latitude on Earth. The equator divides the Earth into the Northern and Southern Hemispheres, called 0 degrees latitude. The other latitude lines are numbered from 0 to 90 degrees toward each pole. The lines north of the equator toward the North Pole are north latitude, and each number is followed by the letter “N.” The lines south of the equator toward the South Pole are south latitude, and the letter “S follows each of the numbers.” The equator (0 latitude) is the only line of latitude without any letter following the number. Notice that all lines of latitude are parallel to the equator (often called parallels) and that the North Pole equals 90 degrees N. The South Pole equals 90 degrees S. Noted parallels include the Tropic of Cancer and the Tropic of Capricorn, 23.5 degrees from the equator. At 66.5 degrees from the equator are the Arctic Circle and the Antarctic Circle near the North and South Pole, respectively. The following are the essential parallel lines:
- Equator, 0 degrees
- Tropic of Cancer, 23.5 degrees north
- Tropic of Capricorn, 23.5 degrees south
- Arctic Circle, 66.5 degrees north
- Antarctic Circle, 66.5 degrees south
- North Pole, 90 degrees north (infinitely small circle)
- South Pole, 90 degrees south (infinitely small circle)
Longitude and Meridians
Longitude is the angular distance of a place east or west of the meridian at Greenwich, England, or west of the standard meridian of a celestial object, usually expressed in degrees and minutes or decimal degrees. Lines connecting points of longitude are called meridians. The prime meridian sits at 0 degrees longitude and divides the Earth into the Eastern and Western Hemispheres. The prime meridian is defined as an imaginary line that runs through the Royal Observatory in Greenwich, England, a suburb of London. The Eastern Hemisphere includes the continents of Europe, Asia, and Australia, while the Western Hemisphere includes North and South America. All meridians (lines of longitude) east of the prime meridian (0 and 180) are numbered from 1 to 180 degrees east (E); the lines west of the prime meridian (0 and 180) are numbered from 1 to 180 degrees west (W). The 0 and 180 lines do not have a letter attached to them. The meridian at 180 degrees is called the International Date Line. The International Date Line (180 degrees longitude) is opposite the prime meridian and indicates the start of each day (Monday, Tuesday, etc.). Each day officially starts at 12:01 a.m. on the International Date Line. Do not confuse the International Date Line with the prime meridian (0 longitude). The actual International Date Line does not follow the 180-degree meridian exactly. Several alterations have been made to the International Date Line to accommodate political agreements to include an island or country on one side of the line or another. Latitude is also sometimes described as zones of latitude. Some of these zones of latitude include:
- Low latitude – between the equator and 30 degrees north
- Midlatitude – between 30 degrees and 60 degrees north and south
- High latitude – latitudes greater than about 60 degrees north and south
- Equatorial – within a few degrees of the equator
- Tropical – within the tropics (between 23.5 degrees north and 23.5 degrees south
- Subtropical – slightly pole-ward of the tropics, around 25-30 degrees north and south
- Polar – within a few degrees of the North Pole or the South Pole
Great and Small Circles

Much of Earth’s grid system is based on the location of the North Pole, South Pole, and the Equator. The poles are an imaginary line running from the axis of Earth’s rotation. The plane of the equator is an imaginary horizontal line that cuts the Earth into two halves. This brings up the topic of great and small circles. A great circle is any circle that divides the Earth into a circumference of two halves. It is also the largest circle that can be drawn on a sphere. The line connecting points along a great circle is also the shortest distance between those two points. Examples of great circles include the Equator, all lines of longitude, the line that divides the Earth into day and night called the circle of illumination, and the ecliptic plane, which divides the Earth into equal halves along the equator. Small circles cut the planet, but not into equal halves. Examples of small circles include all latitude lines except the equator, the Tropical of Cancer, the Tropic of Capricorn, the Arctic Circle, and the Antarctic Circle.
Climate and Latitude
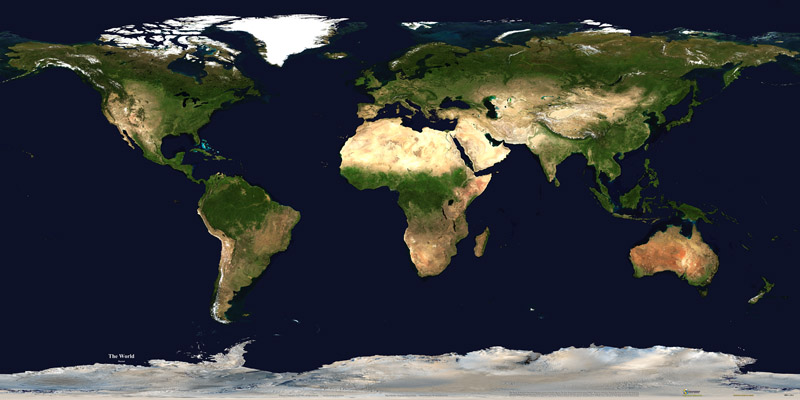
The Earth is tilted on its axis at 23.5 degrees. As it rotates around the sun, the tilt of the Earth’s axis provides different climatic seasons because of the variations in the angle of direct sunlight on the planet. Places receiving more direct sunlight experience a warmer climate. Elsewhere, the increased angle of incoming solar radiation near the Earth’s poles results in more reflected sunlight and a colder climate. The Northern Hemisphere experiences winter when sunlight is reflected off the Earth’s surface, and less of the sun’s energy is absorbed from the sun’s sharper angle.
Time Zones
Universal Time (UT), Coordinated Universal Time (UTC), Greenwich Mean Time (GMT), or Zulu Time (Z): all four terms can be defined as the local time at 0 degrees longitude, which is the prime meridian (location of Greenwich, England). This is the same time under which many military operations, international radio broadcasts, and air traffic control systems operate worldwide. UTC is set in zero- to twenty-four-hour periods instead of two twelve-hour periods (a.m. and p.m.). The designations of a.m. and p.m. are relative to the central meridian: a.m. refers to ante meridiem, or “before noon,” and p.m. refers to post meridiem, or “after-noon.” UT, UTC, GMT, and Z all refer to the same twenty-four-hour time system that unifies a standard time regarding global operations. For example, all air flights use the twenty-four-hour time system so the pilots can coordinate flights across time zones and around the world.
The Earth rotates on its axis once every twenty-four hours at the rate of 15 degrees per hour (15 × 24 = 360). Time zones are established roughly every 15 degrees longitude so that local times correspond to similar hours of day and night. With this system, the sun is overhead at noon in every time zone that follows the 15-degree-wide system. The twenty-four time zones are based on the prime meridian regarding Universal Coordinated Time (UTC), Greenwich Mean Time (GMT), or Zulu Time (Z), which all operate on the twenty-four-hour time clock. Local time zones are either plus or minus, determined by the distance from the prime meridian.

The 15-degree time zone problem is that the zones do not necessarily follow state, regional, or local boundaries. The result is that time zones are seldom precisely 15 degrees wide and usually have various boundary lines. In the United States, the boundaries between the different time zones are inconsistent with the lines of longitude; in some cases, time zones zigzag to follow state lines or to keep cities within a single time zone. Other countries address the problem differently. China, for example, is a significant inland area like the United States yet operates in only one time zone for the entire country.
Coordinate Systems
Just as all maps have a map scale, all maps have locations, too. A geographic coordinate system (GCS) is a spherical or geodetic coordinate system for measuring and communicating positions directly on the Earth, such as latitude and longitude. It is the simplest, oldest, and most widely used spatial reference system and forms the basis for most others1. The definition of a geographic coordinate system includes a datum, prime meridian, and angular unit. The unit of measure in the GCS is degrees, and their respective latitude and longitude define locations within the GCS. Latitude is measured relative to the equator at zero degrees, with a maximum of ninety degrees north at the North Pole or ninety degrees south at the South Pole. Longitude is measured relative to the prime meridian at zero degrees, with a maximum of 180 degrees west or 180 degrees east.
Note that latitude and longitude can be expressed in degrees-minutes-seconds (DMS) or decimal degrees (DD). When using decimal degrees, latitudes above the equator and longitudes east of the prime meridian are positive, and latitudes below the equator and longitudes west of the prime meridian are negative (see the following table for examples). Converting from DMS to DD is a straightforward exercise. For instance, since there are sixty minutes in one degree, we can convert 118° 15 minutes to 118.25 (118 + 15/60). Note that an online search of the term “coordinate conversion” will return several coordinate conversion tools.
When we want to map things like mountains, rivers, streets, and buildings, we need to define how the lines of latitude and longitude will be oriented and positioned on the sphere. A datum serves this purpose and precisely specifies the orientation and origins of the lines of latitude and longitude relative to the center of the Earth or spheroid.
Depending on the need, situation, and location, there are several datums to choose from. For instance, local datums try to match the spheroid closely to the Earth’s surface in a local area and return accurate local coordinates. A typical local datum in the United States is NAD83 (i.e., North American Datum of 1983). For locations in the United States and Canada, NAD83 returns relatively accurate positions, but positional accuracy deteriorates when outside of North America. The global WGS84 datum (i.e., World Geodetic System of 1984) uses the center of the Earth as the origin of the GCS and defines locations across the globe. Because the datum uses the planet’s center as its origin, locational measurements tend to be more consistent regardless of where they are obtained on the planet. However, they may be less accurate than those returned by a local datum. Switching between datums will alter the coordinates (i.e., latitude and longitude) for all locations of interest.
Map Projections
The Earth is not flat or round but a spherical shape. A globe is an excellent representation of the three-dimensional, spheroid Earth. One of the problems with planets, however, is that they are not very portable (i.e., you cannot fold a globe and put it in your pocket), and their small scale makes them of limited practical use (i.e., geographic detail is sacrificed). To overcome these issues, it is necessary to transform the three-dimensional shape of the Earth to a two-dimensional surface like a flat piece of paper, computer screen, or mobile device display to obtain more helpful map forms and map scales. Enter the map projection.
A map projection is a method or a result of transforming the curved surface of a globe into a flat map using a grid of latitude and longitude lines. In cartography, a map projection is a broad set of transformations employed to represent the curved two-dimensional surface of a globe on a plane. In a map projection, coordinates often expressed as latitude and longitude, of locations from the globe’s surface, are transformed into coordinates on a plane.
To illustrate the concept of a map projection, imagine placing a light bulb in the center of a translucent globe. On the world are outlines of the continents and the lines of longitude and latitude called the graticule. When we turn the light bulb on, the outline of the continents and the graticule will be “projected” as shadows on the wall, ceiling, or any other nearby surface. This is what is meant by map “projection.” Within maps and mapping, three surfaces are used for map projections (i.e., surfaces on which we project the shadows of the graticule). These surfaces are the plane, the cylinder, and the cone. Referring to the previous example of a light bulb in the center of a globe, note that we can situate each surface in several ways during the projection process. For example, surfaces can be tangential to the world along the equator or poles, pass through or intersect the surface, and be oriented at any number of angles.
Naming conventions for many map projections include the surface and its orientation. For example, as the name suggests, “planar” projections use the plane, “cylindrical” projections use cylinders, and “conic” projections use the cone. A cylindrical projection is a map projection obtained by projecting points from the globe’s surface onto a cylinder. The cylinder is then unrolled to obtain a flat map. Cylindrical projections are often used for world maps because they are easy to construct and preserve the shape of land masses. Maps representing angles between locations, referred to as bearings, are called conformal. Conformal map projections are used for navigational purposes due to the importance of maintaining a bearing or heading when traveling great distances. The cost of preserving bearings is that areas tend to be quite distorted in conformal map projections. Though shapes are maintained over small areas, areas become wildly distorted at small scales. The Mercator projection (cylindrical) is an example of a conformal projection famous for distorting Greenland.
A conic projection is a map projection obtained by projecting points from the globe’s surface onto a cone. The cone is then unrolled to obtain a flat map. Conic projections are often used for maps of midlatitude zones with east-west orientation because distortion is constant along standard parallels. Finally, a planar projection is a map projection obtained by projecting points from the globe’s surface onto a plane. The plane is then unrolled to obtain a flat map. Planar projections are also known as azimuthal projections or zenithal projections. This type of projection is usually tangent to the globe at one point but may also be second. The point of contact may be the North Pole, the South Pole, a point on the equator, or any point in between.
An equidistant projection is a map projection in which the distances between one or two points and every other point on the map differ from the corresponding distances on the sphere by only a constant scaling factor. The equidistant projection maintains scale along one or more lines or from one or two points to all other points on the map. The azimuthal equidistant projection is an azimuthal map projection that has the valuable properties that all points on the map are at proportionally correct distances from the center point and that all points on the map are at the correct azimuth (direction) from the center point.
As the name indicates, equal-area or equivalent projections preserve the area’s quality. Such projections are particularly useful when accurate measures or comparisons of geographical distributions are necessary (e.g., deforestation, wetlands). To maintain true proportions in the Earth’s surface, features sometimes become compressed or stretched depending on the orientation of the projection. Moreover, such projections distort distances as well as angular relationships.
When moving from the three-dimensional surface of the Earth to a two-dimensional plane, distortions are not only introduced but also inevitable. Map projections introduce distortions in distance, angles, and areas. Depending on the map’s purpose, a series of trade-offs will need to be made concerning such distortions.
As noted earlier, there are theoretically infinite map projections to choose from. One of the key considerations behind the choice of map projection is reducing the distortion. The geographical object being mapped and the respective scale at which the map will be constructed are also essential factors. For instance, maps of the North and South Poles usually use planar or azimuthal projections, and conical projections are best suited for the middle latitude areas of the Earth. Features that stretch east-west, such as the country of Russia, are represented well with the standard cylindrical projection. In contrast, countries oriented north-south (e.g., Chile, Norway) are better described using a transverse projection.
Knowing and understanding map projections are critical within geography and GIS. For instance, to perform an overlay analysis like the one described earlier, all map layers must be at the same point. If not, geographical features will not be appropriately aligned, and any analyses performed will be inaccurate. Most GISs include functions to assist in identifying map projections and transforming between projections to synchronize spatial data. Despite the capabilities of technology, an awareness of the potential and pitfalls surrounding map projections is essential.
Geospatial Technology
Geospatial technology is a term that describes a range of tools that collect, store, analyze, map, and use geographic information that is referenced to the earth1. Geospatial technology can help visualize, simulate, and impact the assessment of human and natural activities1. Geospatial technology includes Geographic Information Systems (GIS), Remote Sensing (RS), Global Positioning System (GPS), and other related technologies that use satellite imagery, aerial photography, machine learning, and spatial thinking.
Global Positioning Systems
Making maps and verifying a location has become more exact with the development of global positioning systems (GPS). The GPS is a United States space-based navigation system that helps pinpoint a three-dimensional position to about a meter of accuracy (for example, latitude, longitude, and altitude) and provides precise nanosecond time anywhere on Earth. GPS comprises three parts: satellites, ground stations, and receivers. Satellites act like stars in constellations—we know where they are supposed to be at any given time. Ground stations use radar to monitor the satellites’ exact positions in space. Receivers, such as navigation devices in cars or boats, use the satellites’ locations in space to pinpoint their location.
GPS is a system of navigation satellites that circle Earth and send signals. A GPS receiver in your phone or any device listens for these signals and calculates its distance from four or more satellites. By doing that, it computes its location in latitude, longitude, and altitude, as well as the accurate time1. GPS includes 24 satellites that circle Earth in precise orbits. Each satellite makes a complete orbit of Earth every 12 hours. These satellites are constantly sending out radio signals. GPS receivers are programmed to receive information about where each satellite is at any given moment2. The Global Positioning System (GPS) is a U.S.-owned utility that provides users with positioning, navigation, and timing (PNT) services. This system consists of three segments: the space segment, the control segment, and the user segment. The U.S. Space Force develops, maintains, and operates the space and control segments.
GPS has many uses, such as finding the exact location of any object or person, assisting in moving from one place to another, monitoring the movement of any object or person, creating maps of any place or part of the world, taking precise time measurements, helping in emergency response systems and predicting the weather, supporting urban development and route optimization, solving positioning problems in sectors such as precision agriculture, automotive, and defense1. GPS technology is now in everything from cell phones and wristwatches to bulldozers, shipping containers, and ATMs. GPS boosts productivity across a wide swath of the economy, including farming, construction, mining, surveying, package delivery, and logistical supply chain management. Major communications networks, banking systems, financial markets, and power grids depend heavily on GPS for precise time synchronization.
Remote Sensing
Remote sensing is the acquisition of information about an object or phenomenon without making physical contact with the object, and thus, it is in contrast to on-site observation. Remote sensing is used in numerous fields, including geography, land surveying, and most Earth science disciplines (for example, hydrology, ecology, meteorology, oceanography, glaciology, geology); it also has military, intelligence, commercial, economic, planning, and humanitarian applications. Remote sensing is the science of obtaining information about objects or areas from a distance. This is done by sensing radiation emitted or reflected by the object or place.
There are two types of remote sensing instruments – active and passive. Active remote sensing requires transmitting coherent electromagnetic waves at a target, and the target can vary from celestial objects or be pointed toward the ground. Active remote sensing instrumentation includes the energy source on which the measurement is based. RADAR is a widely known form of active remote sensing. Active sensors include radio detection and ranging (radar), altimeters, and scatterometers. Most active sensors operate in the microwave band of the electromagnetic spectrum, allowing them to penetrate the atmosphere under most conditions.
Passive remote sensing detects and measures radiation emitted or reflected by the observed object. Passive remote sensing instruments detect natural energy reflected or emitted from the surface or the atmosphere. Examples of passive remote sensing include optical sensors such as cameras and radiometers that detect visible light, infrared radiation, and ultraviolet radiation.
Remote sensing is used in numerous fields and applications, such as geography, land surveying, Earth science disciplines, military, intelligence, commercial, economic, planning, and humanitarian fields. Some examples of remote sensing uses are predicting and assessing crop production and damage, visualizing and analyzing geographic or spatial data with GIS software, and monitoring temperature changes in the oceans1. Remote sensing can be active or passive, depending on whether it uses its source of energy or records natural energy.
Geographic Information Systems
Geographic information systems (GIS) are computer-based systems that collect, store, manipulate, analyze, and display spatial data. GIS technology is used in many fields, such as geography, cartography, remote sensing, land surveying, public health, natural resource management, and urban planning. GIS allows users to visualize and analyze geographic or spatial data to help make better decisions.
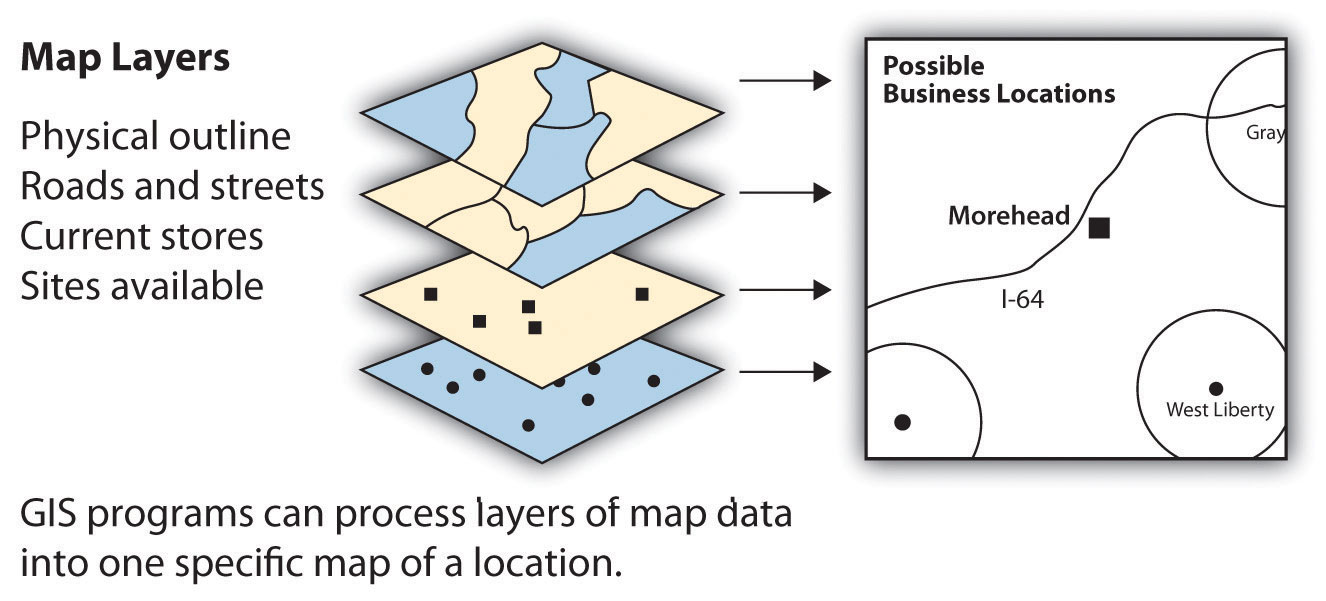
GIS specialists often create and analyze geographical information for government agencies or private businesses. They use computer programs to take raw data to develop the information these organizations need to make vital decisions. For example, in business applications, GIS can determine a desirable location for a retail store based on the analysis of spatial data layers such as population distribution, highway or street arrangements, and the locations of similar stores or competitive establishments. GIS can integrate several maps into one to help analysts understand a place concerning their specific needs.
GIS also focuses on storing information about the Earth (both cultural and natural) in computer databases that can be retrieved and displayed in the form of specialized maps for specific purposes or analyses. GIS specialists require knowledge of computer and database systems. Over the last two decades, GIS has revolutionized the field of cartography: all cartography is now done with the assistance of GIS software. Additionally, analyzing various cultural and natural phenomena through GIS software and specialized maps is integral to urban planning and other social and physical sciences. GIS can also refer to techniques that represent, analyze, and predict spatial relationships between phenomena.