23 Chapter 23: Immune System
Chapter Outline
- 23.1 Introduction to the Immune System
- 23.2 Innate Immunity
- 23.3 Adaptive Immunity
- 23.4 Lymphatic System
- 23.5 Diseases Associated with Suppressed or Overactive Immune Responses

Learning Objectives
After studying this chapter, you should be able to:
- Demonstrate your understanding of the defensive mechanisms of the human body by recognizing (or naming) and describing the components of the three lines of defense and distinguishing the functions of each.
- Understand the range of diseases that affect humans by identifying problems that result from defects in your immune system (i.e., allergy, immunodeficiency, autoimmune disease).
23.1 Introduction to the Immune System
The environment contains numerous pathogens, which are microorganisms that cause diseases in their hosts. A host is the organism that is invaded and often harmed by a pathogen. Pathogens include viruses, bacteria, protists, fungi, and other infectious organisms. We are constantly exposed to pathogens in food and water, on surfaces, and in the air. Mammalian immune systems evolved for protection from such pathogens; they are composed of an extremely diverse array of specialized cells and soluble molecules that coordinate a rapid and flexible defense system capable of providing protection from a majority of these disease agents.
Components of the immune system constantly search the body for signs of pathogens. When pathogens are found, immune factors are mobilized to the site of an infection. The immune factors identify the nature of the pathogen, strengthen the corresponding cells and molecules to combat it efficiently, and then halt the immune response after the infection is cleared to avoid unnecessary host cell damage. The immune system can remember pathogens to which it has been exposed to create a more efficient response upon re-exposure. This memory can last several decades. Features of the immune system, such as pathogen identification, specific response, amplification, retreat, and remembrance are essential for survival against pathogens. The immune response can be classified as either innate or adaptive. The innate immune response is always present and attempts to defend against all pathogens rather than focusing on specific ones. Conversely, the adaptive immune response remembers past infections and mounts pathogen-specific defenses.
23.2 Innate Immunity
The human immune system is a complex, multilayered system for defending against external and internal threats to the integrity of the body. The system can be divided into two types of defense systems: the innate immune system, which is nonspecific toward a particular kind of pathogen, and the adaptive immune system, which is specific (Figure 23.2). Innate immunity is not caused by an infection or vaccination and depends initially on physical and chemical barriers that work on all pathogens, sometimes called the first line of defense. The second line of defense of the innate system includes chemical signals that produce inflammation and fever responses as well as mobilizing protective cells and other chemical defenses. The adaptive immune system mounts a highly specific response to substances and organisms that do not belong in the body. The adaptive system takes longer to respond and has a memory system that allows it to respond with greater intensity should the body reencounter a pathogen even years later.

External and Chemical Barriers
The body has significant physical barriers to potential pathogens. The skin contains the protein keratin, which resists physical entry into cells. Other body surfaces, particularly those associated with body openings, are protected by the mucous membranes. The sticky mucus provides a physical trap for pathogens, preventing their movement deeper into the body. The openings of the body, such as the nose and ears, are protected by hairs that catch pathogens, and the mucous membranes of the upper respiratory tract have cilia that constantly move pathogens trapped in the mucus coat up to the mouth.
The skin and mucous membranes also create a chemical environment that is hostile to many microorganisms. The surface of the skin is acidic, which prevents bacterial growth. Saliva, mucus, and the tears of the eye contain an enzyme that breaks down bacterial cell walls. The stomach secretions create a highly acidic environment, which kills many pathogens entering the digestive system.
Finally, the surface of the body and the lower digestive system have a community of microorganisms such as bacteria, archaea, and fungi that coexist without harming the body. There is evidence that these organisms are highly beneficial to their host, combating disease-causing organisms and outcompeting them for nutritional resources provided by the host body. Despite these defenses, pathogens may enter the body through skin abrasions or punctures, or by collecting on mucosal surfaces in large numbers that overcome the protections of mucus or cilia.
Internal Defenses
When pathogens enter the body, the innate immune system responds with a variety of internal defenses. These include the inflammatory response, phagocytosis, natural killer cells, and the complement system. White blood cells in the blood and lymph recognize pathogens as foreign to the body. A white blood cell is larger than a red blood cell, is nucleated, and is typically able to move using amoeboid locomotion. Because they can move on their own, white blood cells can leave the blood to go to infected tissues. For example, a monocyte is a type of white blood cell that circulates in the blood and lymph and develops into a macrophage after it moves into infected tissue. A macrophage is a large cell that engulfs foreign particles and pathogens.
When a pathogen is recognized as foreign, chemicals called cytokines are released. A cytokine is a chemical messenger that regulates cell differentiation (type and function), proliferation (production), and gene expression to produce a variety of immune responses. Approximately 40 types of cytokines exist in humans. In addition to being released from white blood cells after pathogen recognition, cytokines are also released by the infected cells and bind to nearby uninfected cells, inducing those cells to release cytokines. This positive feedback loop results in a burst of cytokine production.
One class of early-acting cytokines is the interferons, which are released by virus infected cells as a warning to nearby uninfected cells. An interferon is a small protein that signals a viral infection to other cells. The interferons stimulate uninfected cells to produce compounds that interfere with viral replication. Interferons also activate macrophages and other cells.
The Inflammatory Response and Phagocytosis
The first cytokines to be produced encourage inflammation, a localized reaction that produces redness, swelling, heat, and pain. Inflammation is a response to physical trauma, such as a cut or a blow, chemical irritation, and infection by pathogens (viruses, bacteria, protists, or fungi). The chemical signals that trigger an inflammatory response enter the extracellular fluid and cause capillaries to dilate (expand) and capillary walls to become more permeable, or leaky. The serum and other compounds leaking from capillaries cause swelling of the area, which in turn causes pain. Various kinds of white blood cells are attracted to the area of inflammation. The types of white blood cells that arrive at an inflamed site depend on the nature of the injury or infecting pathogen. For example, a neutrophil is an early arriving white blood cell that engulfs and digests pathogens. Neutrophils are the most abundant white blood cells of the immune system (Figure 23.3). Macrophages follow neutrophils and take over the phagocytosis function and are involved in the resolution of an inflamed site, cleaning up cell debris and pathogens.

Cytokines also send feedback to cells of the nervous system to bring about the overall symptoms of feeling sick, which include lethargy, muscle pain, and nausea. Cytokines also increase the core body temperature, causing a fever. The elevated temperatures of a fever inhibit the growth of pathogens and speed up cellular repair processes. For these reasons, suppression of fevers should be limited to those that are dangerously high.
Concept in Action
Check out this 23-second, stop-motion video showing a neutrophil that searches and engulfs fungus spores during an elapsed time of 79 minutes.
Natural Killer Cells
A lymphocyte is a white blood cell that contains a large nucleus (Figure 23.4). Most lymphocytes are associated with the adaptive immune response, but infected cells are identified and destroyed by natural killer cells, the only lymphocytes of the innate immune system. A natural killer (NK) cell is a lymphocyte that can kill cells infected with viruses or cancerous cells. NK cells identify intracellular infections, especially from viruses, by the altered expression of major histocompatibility class (MHC) I molecules on the surface of infected cells. MHC class I molecules are proteins on the surfaces of all nucleated cells that provide a sample of the cell’s internal environment at any given time. Unhealthy cells, whether infected or cancerous, display an altered MHC class I complement on their cell surfaces.

After the NK cell detects an infected cell or tumor cell, it causes the cell to commit suicide (also called programmed cell death or apoptosis). Phagocytic cells then come along and digest the cell debris left behind. NK cells are constantly patrolling the body and are an effective mechanism for controlling potential infections and preventing cancer progression. The various types of immune cells are shown in Figure 23.5.

Complement System
An array of approximately 20 types of proteins, called the complement system, is also activated by infection or the activity of the cells of the adaptive immune system and functions to destroy extracellular pathogens. Liver cells and macrophages synthesize inactive forms of complement proteins continuously; these proteins are abundant in the blood and are capable of responding immediately to infecting microorganisms. The complement system is so named because it is complementary to the innate and adaptive immune system. Complement proteins bind to the surfaces of microorganisms and are particularly attracted to pathogens that are already tagged by the adaptive immune system. This “tagging” involves the attachment of specific proteins called antibodies (discussed in detail later) to the pathogen. When they attach, the antibodies change shape providing a binding site for one of the complement proteins. After the first few complement proteins bind, a cascade of binding in a specific sequence of proteins follows in which the pathogen rapidly becomes coated in complement proteins.
Complement proteins perform several functions, one of which is to serve as a marker to indicate the presence of a pathogen to phagocytic cells and enhance engulfment. Certain complement proteins can combine to open pores in microbial cell membranes and cause lysis of the cells.
Section Summary
The innate immune system consists first of physical and chemical barriers to infection including the skin and mucous membranes and their secretions, ciliated surfaces, and body hairs. The second line of defense is an internal defense system designed to counter pathogenic threats that bypass the physical and chemical barriers of the body. Using a combination of cellular and molecular responses, the innate immune system identifies the nature of a pathogen and responds with inflammation, phagocytosis, cytokine release, destruction by NK cells, or the complement system.
23.3 Adaptive Immunity
The adaptive, or acquired, immune response takes days or even weeks to become established—much longer than the innate response; however, adaptive immunity is more specific to an invading pathogen. Adaptive immunity is an immunity that occurs after exposure to an antigen either from a pathogen or a vaccination. An antigen is a molecule that stimulates a response in the immune system. This part of the immune system is activated when the innate immune response is insufficient to control an infection. In fact, without information from the innate immune system, the adaptive response could not be mobilized. There are two types of adaptive responses: the cell-mediated immune response, which is controlled by activated T cells, and the humoral immune response, which is controlled by activated B cells and antibodies. Activated T and B cells whose surface binding sites are specific to the molecules on the pathogen greatly increase in numbers and attack the invading pathogen. Their attack can kill pathogens directly or they can secrete antibodies that enhance the phagocytosis of pathogens and disrupt the infection. Adaptive immunity also involves a memory to give the host long-term protection from reinfection with the same type of pathogen; on re-exposure, this host memory will facilitate a rapid and powerful response.
B and T Cells
Lymphocytes, which are white blood cells, are formed with other blood cells in the red bone marrow found in many flat bones, such as the shoulder or pelvic bones. The two types of lymphocytes of the adaptive immune response are B and T cells (Figure). Whether an immature lymphocyte becomes a B cell or T cell depends on where in the body it matures. The B cells remain in the bone marrow to mature (hence the name “B” for “bone marrow”), while T cells migrate to the thymus (located above the heart), where they mature (hence the name “T” for “thymus”).
Maturation of a B or T cell involves becoming immunocompetent, meaning that it can recognize, by binding, a specific molecule or antigen (discussed below). During the maturation process, B and T cells that bind too strongly to the body’s own cells are eliminated in order to minimize an immune response against the body’s own tissues. Those cells that react weakly to the body’s own cells, but have highly specific receptors on their cell surfaces that allow them to recognize a foreign molecule, or antigen, remain. This process occurs during fetal development and continues throughout life. The specificity of this receptor is determined by the genetics of the individual and is present before a foreign molecule is introduced to the body or encountered. Thus, it is genetics and not experience that initially provides a vast array of cells, each capable of binding to a different specific foreign molecule. Once they are immunocompetent, the T and B cells will migrate to the spleen and lymph nodes where they will remain until they are called on during an infection. B cells are involved in the humoral immune response (humor is the old term for body fluid), which targets pathogens loose in body fluids (blood and lymph), and T cells (Figure 23.6) are involved in the cell-mediated immune response, which targets infected cells and cancer cells.

Humoral (or Antibody)-Mediated Immune Response
As mentioned, an antigen is a molecule that stimulates a response in the immune system. Not every molecule is antigenic. B cells participate in a chemical response to antigens present in the body by producing specific antibodies that circulate throughout the body in the body fluids and bind with the antigen whenever it is encountered. This is known as the humoral immune response. As discussed, during maturation of B cells, many different highly specific B cells are produced that each recognize a different antigen with the receptor molecules in their membrane (Figure 23.7).

Each B cell has only one kind of antigen receptor, which makes every B cell different. Once the B cells mature in the bone marrow, they migrate to lymph nodes or other lymphatic organs. When a B cell encounters the antigen that binds to its receptor, the antigen molecule is brought into the cell by endocytosis and reappears on the surface of the cell bound to an MHC class II molecule. When this process is complete, the B cell is sensitized. In most cases, the sensitized B cell must then encounter a specific kind of T cell, called a helper T cell, before it is activated. The helper T cell must already have been activated through an encounter with the antigen (discussed below).
The helper T cell binds to the antigen-MHC class II complex and is stimulated to release cytokines that cause the B cell to divide rapidly, which makes thousands of identical (clonal) cells via mitosis. This process is called clonal expansion. These daughter cells become either plasma cells or memory B cells. The memory B cells remain inactive at this point until another later encounter with the antigen caused by a reinfection by the same bacteria or virus results in them dividing into a new population of plasma cells. The plasma cells, on the other hand, produce and secrete large quantities, up to 100 million molecules per hour, of antibody molecules. An antibody, also known as an immunoglobulin (Ig), is a protein that is produced by plasma cells after stimulation by an antigen. Antibodies are the agents of humoral immunity. Antibodies occur in the blood, in gastric and mucus secretions, and in breast milk. Antibodies in these bodily fluids can bind pathogens and mark them for destruction by phagocytes and make it easier for the phagocytes to ingest them (called opsonization) before they can infect cells. Antibodies can also neutralize the pathogen by blocking it from binding to our cells (called neutralization). And finally, antibodies can activate the complement system, which will get rid of the pathogen (see Figure 23.8).
Figure 23.8 Antibodies may inhibit infection by (a) preventing the antigen from binding its target, (b) tagging a pathogen for destruction by macrophages or neutrophils, or (c) activating the complement cascade. (credit: Openstax Human Biology)
The production of antibodies by plasma cells in response to an antigen is called active immunity and describes the host’s active response of the immune system to an infection or to a vaccination. In passive immunity, antibodies come from an outside source, instead of the individual’s own plasma cells, and are introduced into the host. For example, antibodies circulating in a pregnant woman’s body move across the placenta into the developing fetus. Mom’s antibodies are also passed to the baby after birth in the colostrum, the first milk. The child benefits from the presence of these antibodies for up to several months after birth. Another example of passive immunity is injecting antibodies into an individual in the form of an antivenom to a snake-bite toxin or antibodies in blood serum to help fight a hepatitis infection. This gives immediate protection since the body does not need the time required to mount its own response.
Cell-Mediated Immunity
Unlike B cells, T lymphocytes are unable to recognize pathogens without assistance. Instead, dendritic cells (a type of white blood cell found in the tissues) and macrophages first engulf and digest pathogens into hundreds or thousands of antigens. These two cell types are called antigen-presenting cells (APCs) because they then put the antigen fragments bound to MHC class II molecules on their surface and present the antigen to helper T cells (Figure 23.9). Helper T cells cannot properly respond to an antigen unless it is processed and embedded in an MHC class II molecule.

Concept in Action
View this animation from Rockefeller University to see how dendritic cells act as sentinels in the body’s immune system.
There are two main types of T cells: helper T lymphocytes (TH) and the cytotoxic T lymphocytes (TC). The TH lymphocytes function indirectly and help activate B cells and cytotoxic T cells to go after the pathogen that they recognize (Figure 23.10).
Figure 23.10 A helper T cell becomes activated by binding to an antigen presented by an APC via the MHC II receptor, causing it to release cytokines. Depending on the cytokines released, this activates either the humoral or the cell-mediated immune response. (credit: Openstax Human Biology)
Cytotoxic T cells (TC) are the key component of the cell-mediated part of the adaptive immune system and attack and destroy infected cells and cancer cells. TC cells are particularly important in protecting against viral infections; this is because viruses replicate within cells where they are shielded from contact with circulating antibodies outside of the cells. Once activated, the TC creates a large clone of cells with one specific set of cell-surface receptors, as in the case with clonal expansion of activated B cells. As with B cells, the clone includes active TC cells and inactive memory TC cells. The resulting active TC cells then identify infected host cells and kill them. Because of the time required to generate a population of clonal T and B cells, there is a delay in the adaptive immune response compared to the innate immune response.
Plasma cells (activated B cells that produce antibodies) and TC cells are collectively called effector cells because they are involved in “effecting” (bringing about) the immune response of killing pathogens and infected host cells.
Immunological Memory
The adaptive immune response has a memory component that allows for a rapid and large response upon re-invasion of the same pathogen. During the adaptive immune response to a pathogen that has not been encountered before, known as the primary immune response, plasma cells secreting antibodies and differentiated T cells increase, then plateau over time. As B and T cells mature into effector cells, a subset of the naïve populations differentiates into B and T memory cells with the same antigen specificities (Figure 23.11). A memory cell is an antigen-specific B or T lymphocyte that does not differentiate into an effector cell during the primary immune response, but that can immediately become an effector cell on re-exposure to the same pathogen. As the infection is cleared and pathogenic stimuli subside, the effectors are no longer needed and they die off. In contrast, the memory cells persist in the circulation.

If the pathogen is never encountered again during the individual’s lifetime, B and T memory cells will circulate for a few years or even several decades and will gradually die off, having never functioned as effector cells. However, if the host is re-exposed to the same pathogen type, circulating memory cells will immediately differentiate into plasma cells and TC cells without input from APCs or TH cells. This is known as the secondary immune response. One reason why the adaptive immune response is delayed is because it takes time for naïve B and T cells with the appropriate antigen specificities to be identified, activated, and proliferate. On reinfection, this step is skipped, and the result is a more rapid production of immune defenses. Memory B cells that differentiate into plasma cells output tens to hundreds-fold greater antibody amounts than were secreted during the primary response (Figure 3.12). This rapid and dramatic antibody response may stop the infection before it can even become established, and the individual may not realize they had been exposed.

Vaccination is based on the knowledge that exposure to noninfectious antigens, derived from known pathogens, generates a mild primary immune response. The immune response to vaccination may not be perceived by the host as illness but still confers immune memory. When exposed to the corresponding pathogen to which an individual was vaccinated, the reaction is similar to a secondary exposure. Because each re-infection generates more memory cells and increased resistance to the pathogen, some vaccine courses involve one or more booster vaccinations to mimic repeat exposures.
Section Summary
The adaptive immune response is a slower-acting, longer-lasting, and more specific response than the innate response. However, the adaptive response requires information from the innate immune system to function. APCs display antigens on MHC molecules to naïve helper T cells. In response, the helper T cells activate the B cells and cytotoxic T cells that recognize that pathogen. B cells differentiate into plasma cells that secrete antibodies, whereas TC cells destroy infected or cancerous cells. Memory cells are produced by activated and proliferating B and T cells and persist after a primary exposure to a pathogen. If re-exposure occurs, memory cells differentiate into effector cells without input from the innate immune system.
23.4 The Lymphatic System
The immune system is closely tied to the lymphatic system because white blood cells are made in the bone marrow of the lymphatic system and travel through lymphatic vessels and hang out in lymph nodes and other lymphoid organs. The lymphatic system is made up of lymphatic vessels, lymph nodes, and organs, such as tonsils, adenoids, thymus, and spleen. Lymph is the watery fluid that bathes tissues and organs and travels through the lymphatic vessels. It contains protective white blood cells but does not contain red blood cells.
Recall that cells of the immune system originate from stem cells in the bone marrow. B cell maturation occurs in the bone marrow, whereas T cells mature in the thymus.
On maturation, T and B lymphocytes circulate to various destinations through the blood vessels and lymphatic vessels. Lymph nodes scattered throughout the body house large populations of T and B cells, dendritic cells, and macrophages (Figure 23.13). As lymph drains from tissues, it also carries any antigens from pathogens with it. These antigens then are filtered through lymph nodes before the lymph is returned to circulation. APCs in the lymph nodes capture and process antigens and inform nearby helper T lymphocytes about potential pathogens.

The spleen also houses B and T cells along, macrophages, dendritic cells, and NK cells (Figure 23.14). The spleen filters the blood, and these white blood cells look for pathogens in the blood and respond if they find any.

23.5 Diseases Associated with Suppressed or Overactive Immune Responses
This section is about how the immune system goes wrong. When it goes haywire and becomes too weak or too strong, it leads to a state of disease. The factors that maintain immunological homeostasis are complex and incompletely understood.
Immunodeficiencies
As you have seen, the immune system is quite complex. It has many pathways using many cell types and signals. Because it is so complex, there are many ways for it to go wrong. In immunodeficiencies, the immune system does not work well and is too weak to protect the person from pathogens. Inherited immunodeficiencies arise from gene mutations that affect specific components of the immune response. There are also acquired immunodeficiencies with potentially devastating effects on the immune system, such as HIV.
Inherited Immunodeficiencies
One example of an inherited immunodeficiency is severe combined immunodeficiency disease (SCID). Genetic mutations affect both the B cells and T cells of the adaptive immune response and neither work well. Children with this disease usually die of opportunistic infections within their first year of life unless they receive a bone marrow transplant. Such a procedure had not yet been perfected for David Vetter, the “boy in the bubble,” who was treated for SCID by having to live almost his entire life in a sterile plastic cocoon for the 12 years before his death from infection in 1984. One of the features that make bone marrow transplants work as well as they do is the proliferative capability of hematopoietic stem cells of the bone marrow. Only a small amount of bone marrow from a healthy donor is given intravenously to the recipient. It finds its own way to the bone where it populates it, eventually reconstituting the patient’s immune system, which is usually destroyed beforehand by treatment with radiation or chemotherapeutic drugs.
New treatments for SCID using gene therapy, inserting non-defective genes into cells taken from the patient and giving them back, have the advantage of not needing the tissue match required for standard transplants. Although not a standard treatment, this approach holds promise, especially for those in whom standard bone marrow transplantation has failed.
Human Immunodeficiency Virus/AIDS
Although many viruses cause suppression of the immune system, only one wipes it out completely, and that is HIV. The virus is transmitted through semen, vaginal fluids, and blood, and can be caught by risky sexual behaviors and the sharing of needles by intravenous drug users. HIV infects helper T cells and slowly kills them off. With too few helper T cells around, the B cells and cytotoxic T cells cannot be activated to fight off pathogens and cancerous cells. This eventually leads to the development years later of AIDS (acquired immunodeficiency syndrome), where the person gets opportunistic infections with microorganisms that normally do not make healthy people sick and cancers.
Treatment for the disease consists of anti-viral drugs that target the virus. Scientists have been working on an HIV vaccine for 30 years, but one is still years away. Because the virus mutates rapidly to evade the immune system, scientists have been looking for parts of the virus that do not change and thus would be good targets for a vaccine candidate.
Overactive Immune Responses
The immune system can also cause problems by overreacting and responding to the wrong things or by reacting too strongly. We will look at two examples of this: allergies and autoimmune diseases.
Allergies
In allergies, the immune system reacts to substances that are not pathogens and are not harmful to us. Antigens that cause allergic responses are often referred to as allergens. Examples of allergens include pollen, grasses, foods, insect stings, latex, and medications (ex- penicillin). Exposure to the allergen causes certain white blood cells to release the chemical, histamine. Histamine causes the symptoms of allergies. Symptoms of an allergic response include hay fever, asthma, eczema, hives, GI upset, and in severe cases, anaphylactic shock, which can be fatal if not treated. Anaphylactic shock can kill a person because the blood pressure drops (shock) and the airways constrict, making it difficult to breathe. Epinephrine is given to treat anaphylactic shock because it raises blood pressure and opens the airways. Patients with known severe allergies are encouraged to keep automatic epinephrine injectors with them at all times, especially when away from easy access to hospitals.
Allergists use skin testing to identify allergens. In skin testing, allergen extracts are injected into the epidermis, and a positive result of a soft, pale swelling at the site surrounded by a red zone (called the wheal and flare response), caused by the release of histamine, usually occurs within 30 minutes.
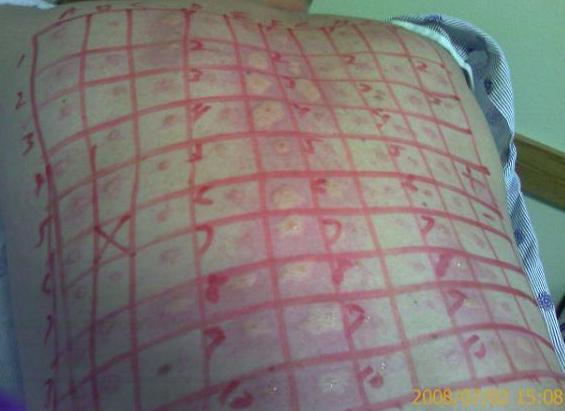
Autoimmune Diseases
In people with an autoimmune disease, the immune system overreacts and attacks their own bodies, causing significant damage. The trigger for these diseases is, more often than not, unknown, and the treatments are usually based on resolving the symptoms using immunosuppressive and anti-inflammatory drugs such as steroids. These diseases can be localized and crippling, as in rheumatoid arthritis, or diffuse in the body with multiple symptoms that differ in different individuals, as is the case with systemic lupus erythematosus (Figure 23.16).
Media Attributions
- Skintest2