10 Chapter 10: Structure Determines Function
Learning Objectives
Learning objectives for the structure and function bioconcept.
- You will be able to demonstrate and provide examples of the intimate relationship between structure (shape) and function in nature at several levels:
- molecular and cellular (proteins and cell types)
- individual (anatomy and physiology)
- population level and above (ecosystems)
- Distinguish among the basic structures and functions of the four tissue types by:
- providing or recognizing major examples of each tissue type
- summarizing how the tissues are organized into organs and systems
- List the 11 organ systems, their components, and their functions.
- Explain how and why organisms must maintain homeostasis within their internal environment.
10.1 Structure Determines Function
One of the overarching themes of biology is that structure determines function; how something is arranged allows it to perform a specific job. We see this at all levels in the hierarchy of biological organization from atoms up to the biosphere. Let’s take a look at some examples where structure determines function.
- Molecular level – proteins. The shape (structure) of a protein determines its function. For example, there are two basic shapes for proteins: fibrous and globular (round). Fibrous proteins, such as collagen (Figure 18.1), are shaped like a rope and give strength to our skin to prevent it from tearing. Fibrous proteins are structural proteins because they help give shape to and support the skin. Globular proteins, such as hemoglobin (Figure 18.2), are used to transport oxygen in the blood. Other examples of globular proteins that have different functions are enzymes (catalyze or speed up chemical reactions in the body) and plasma membrane proteins (can transport substances across the cell membrane, play a role in cell communication, act as enzymes, or help identify the cell to the rest of the body).
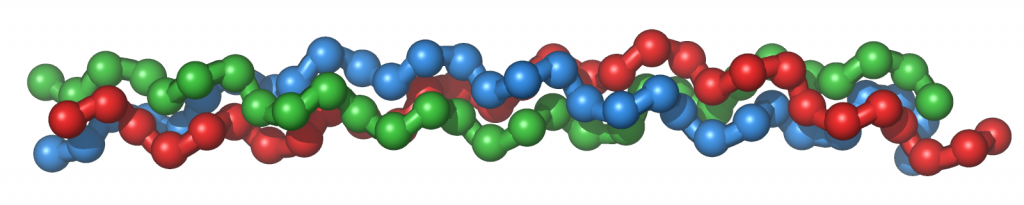
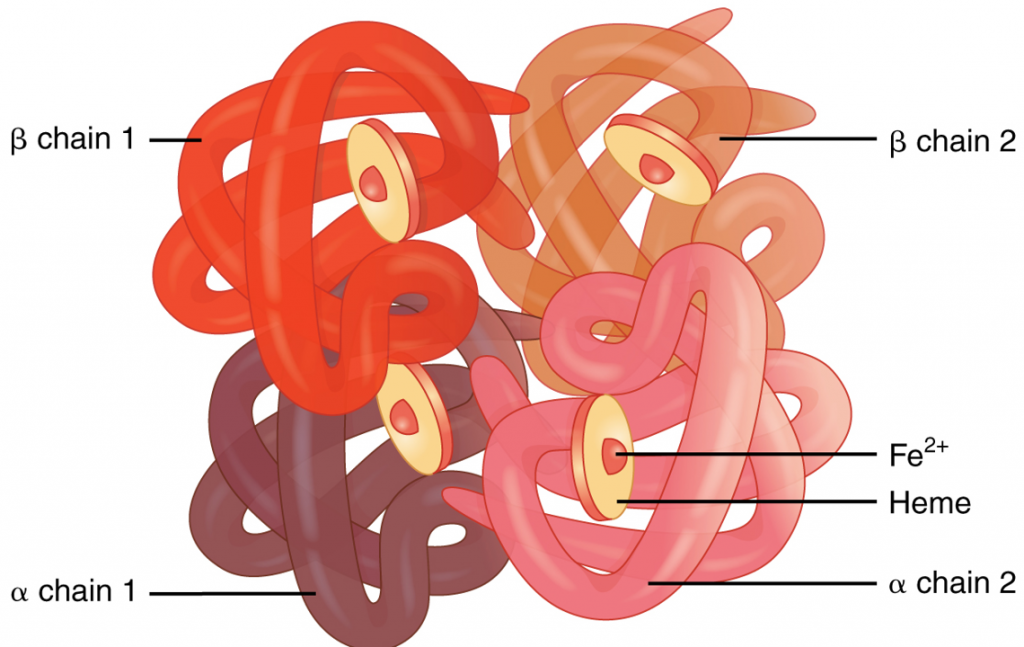
- Cellular level – skeletal muscle cells. The structure of skeletal muscle cells allows them to have the function of contraction, which allows us to move. For example, skeletal muscle cells that make up your biceps brachii muscle are attached to both ends of the humerus bone by tendons and are packed full of contractile proteins (actin and myosin) (Figure 18.3). When the contractile proteins contract, they shorten the muscle cell, which then pulls on the ends of the humerus and allows you to flex your forearm (Figure 18.4).
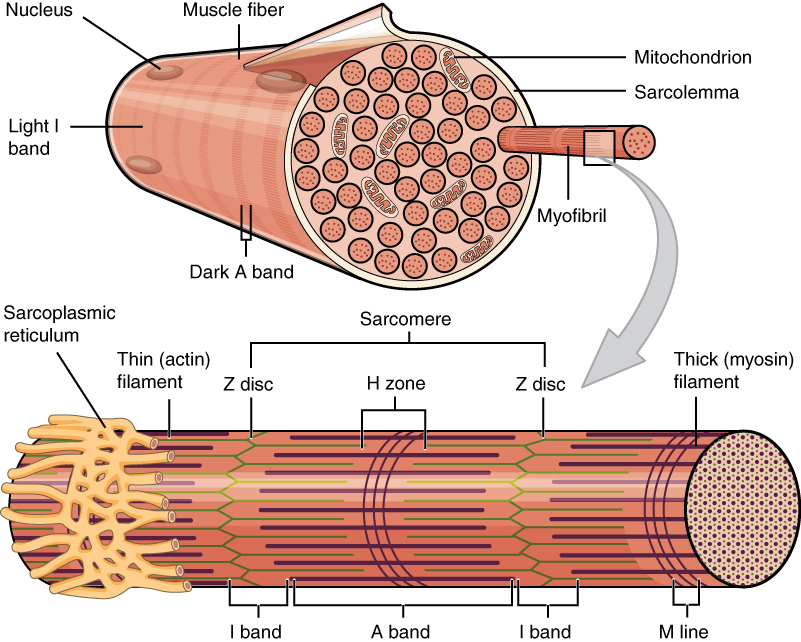
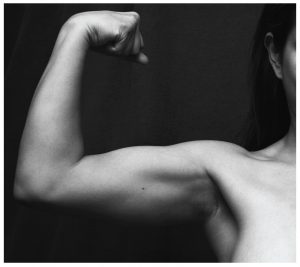
- Individual level (anatomy and physiology). In studying humans, anatomy is the study of the structure of the body (ex- where the quadriceps muscle is located) and physiology is the study of how the body functions (ex- how the quadriceps muscle contracts). Let’s take a look at the anatomy of the heart, which dictates the heart’s function. The heart consists of four hollow chambers (atria and ventricles) and is made of cardiac muscle cells (Figure 18.5). This structure allows the heart to have the function of pumping blood around the body. If the structure of the heart changes (ex- some of the heart chambers become stretched out or dilated), then the heart’s function decreases as the heart can no longer pump as much blood, which will eventually cause congestive heart failure.
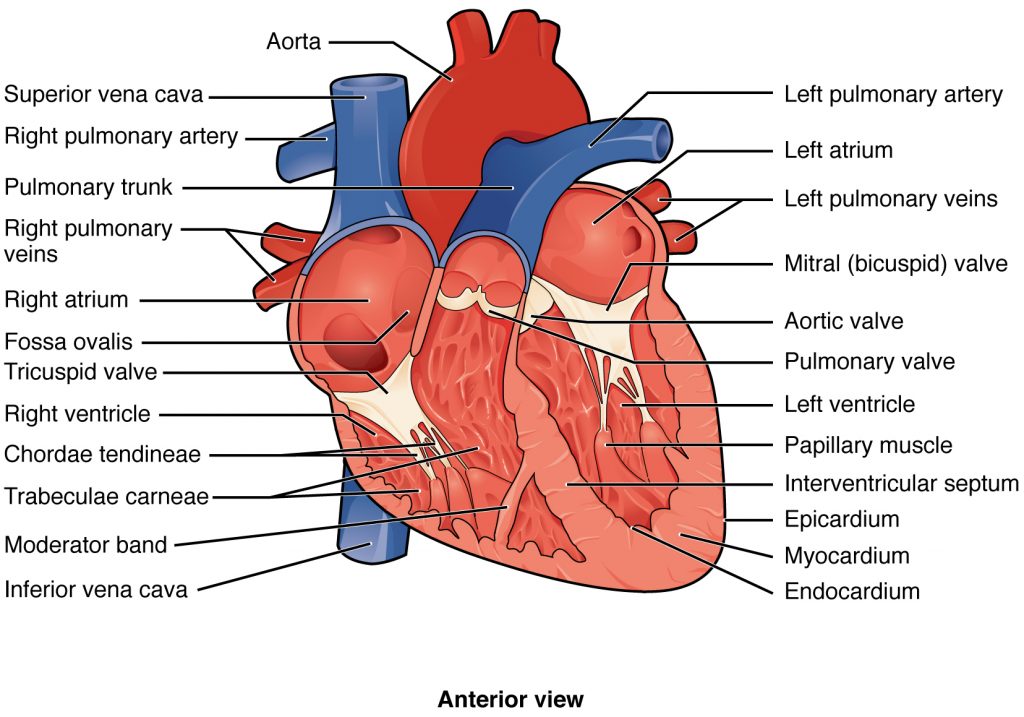
- Ecosystem level. An ecosystem consists of a community of all the different species living in a particular geographic area as well as all of the nonliving components (ex- water, sand, light, oxygen). If we look at the structure of a coral reef ecosystem, we see that the corals, which are the foundation species, provide protection and habitat for other species (Figure 18.6). The coral reef protects other species, such as fish, from ocean waves and currents and gives them a place to hide from predators.
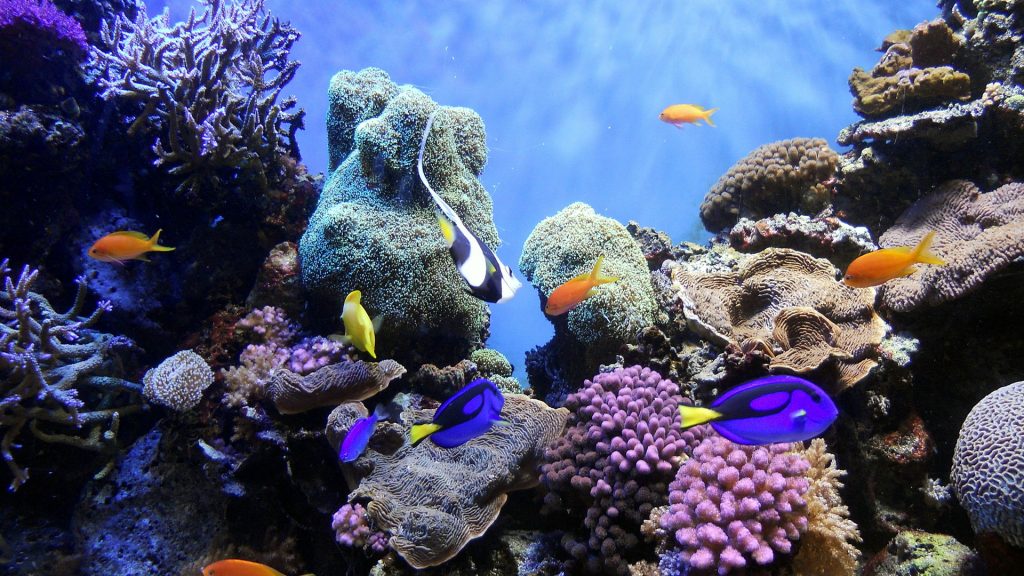
10.2 Human Tissue Types
The term tissue is used to describe a group of similar cells found together in the body that act together to perform specific functions. From the evolutionary perspective, tissues appear in more complex organisms.
Although there are many types of cells in the human body, they are organized into four categories of tissues: epithelial, connective, muscle, and nervous. Each of these categories is characterized by specific functions that contribute to the overall health and maintenance of the body. A disruption of the structure of a tissue is a sign of injury or disease. Such changes can be detected through histology, the microscopic study of tissue appearance, organization, and function.
The Four Types of Tissues
Epithelial tissue, also referred to as epithelium, refers to the sheets of cells that cover exterior surfaces of the body, line internal cavities and passageways, and form certain glands. Examples of epithelial tissue include skin, mucous membranes, endocrine glands, and sweat glands. Connective tissue, as its name implies, binds the cells and organs of the body together and functions in the protection, support, and integration of all parts of the body. Connective tissue is diverse and includes bone, tendons, ligaments, cartilage, fat, and blood. Muscle tissue is excitable, responding to stimulation and contracting to provide movement, and occurs as three major types: skeletal (voluntary) muscle, smooth muscle, and cardiac muscle in the heart. Nervous tissue is also excitable, allowing the propagation of electrochemical signals in the form of nerve impulses that communicate between different regions of the body (Figure 18.7).
The next level of organization is the organ, where two or more types of tissues come together to perform specific functions. Just as knowing the structure and function of cells helps you in your study of tissues, knowledge of tissues will help you understand how organs function.
10.3 Human Organ Systems
An organ system is a group of organs that work together to perform major functions or meet physiological needs of the body. Figure 18.8 below shows the eleven distinct organ systems in the human body. Assigning organs to organ systems can be imprecise since organs that “belong” to one system can also have functions integral to another system. In fact, most organs contribute to more than one system. In this course, we will discuss some, but not all of these organ systems.
.jpg)
Table 10.1 below lists the 11 organ systems, their components, and functions.
|
||||||||||||||||||||||||||||||||||||||
10.4 Homeostasis
Before moving on to discussing the individual organ systems, it is important to review the concept of homeostasis. Homeostasis refers to the maintenance of a relatively stable state inside the body. Human organs and organ systems constantly adjust to internal and external changes in order to maintain this steady state. Examples of internal conditions maintained in homeostasis are the level of blood glucose, body temperature, and blood calcium level. These conditions remain stable because of control by negative feedback. If the blood glucose or calcium rises, this sends a signal to organs responsible for lowering blood glucose or calcium. The signals that restore the variable to the normal range (also called the set point) are examples of negative feedback. When homeostatic mechanisms fail, the person gets sick and could die.
Control of Homeostasis
When a change occurs in an person’s environment, an adjustment must be made. A receptor (often a neuron) senses the change in the environment, then sends a signal to the control center (in most cases, the brain) which in turn generates a response that is signaled to an effector, which returns the regulated variable back to the normal range. The effector is a muscle (that contracts or relaxes) or a gland that secretes. Homeostatsis is maintained by negative feedback loops. Positive feedback loops actually push the organism further out of homeostasis, but may be necessary for life to occur. Homeostasis is controlled by the nervous and endocrine systems.
Negative Feedback Mechanisms
Any homeostatic process that changes the direction of the stimulus back toward the normal range is a negative feedback loop. It may either increase or decrease the stimulus, but the stimulus is not allowed to continue as it did before the receptor sensed it. In other words, if a level is too high, the body does something to bring it down, and conversely, if a level is too low, the body does something to make it go up. Hence the term negative feedback. An example is the maintenance of blood glucose levels. After a person has eaten, blood glucose levels rise. Specialized cells in the pancreas sense this, and the hormone insulin is released by the endocrine system. Insulin causes blood glucose levels to decrease, as would be expected in a negative feedback system, as illustrated in Figure 18.9. However, if a person has not eaten and blood glucose levels decrease, this is sensed in another group of cells in the pancreas, and the hormone glucagon is released causing glucose levels to increase. This is still a negative feedback loop, but not in the direction expected by the use of the term “negative.” Negative feedback loops are the predominant mechanism used to maintain homeostasis.
Thermoregulation
Another example of the use of negative feedback to maintain homeostasis is thermoregulation. Animals, such as humans, that maintain a constant body temperature in the face of differing environmental temperatures, are called endotherms. We are able to maintain this temperature by generating internal heat (a waste product of the cellular chemical reactions of metabolism) that keeps the cellular processes operating optimally even when the environment is cold.
Thermoreceptors (made of neurons) in the internal organs, spine, and brain send information about the body temperature to the control center in the hypothalamus in the brain. The hypothalamus acts as the body’s thermostat and can raise or lower the body temperature to keep it in the normal range (around 98.6 ºF or 37 ºC). If the body temperature is above the normal range, the hypothalamus will send signals to the sweat glands to cause sweating and to the smooth muscle around the blood vessels in the skin to cause vasodilation. Vasodilation, the opening up of arteries to the skin by relaxation of their smooth muscles, brings more blood and heat to the body surface, facilitating heat loss and cooling the body. Conversely, if the body temperature is below the normal range, the hypothalamus will tell the skeletal muscles to contract to cause shivering, which will generate body heat. Signals are also sent to the smooth muscle around the blood vessels in the skin to cause vasoconstriction. Vasoconstriction, the narrowing of blood vessels to the skin by contraction of their smooth muscles, reduces blood flow in peripheral blood vessels, forcing blood toward the core and vital organs, conserving heat.
The normal range (set point) for body temperature may be changed during an infection. Some of your immune system cells release chemicals called pyrogens, which cause the hypothalamus to reset the body temperature normal range to a higher value, resulting in a fever. The increase in body heat makes the body less optimal for bacterial growth and increases the activities of immune system cells so they are better able to fight the infection.

Positive Feedback
A positive feedback loop pushes the regulated variable further away from the normal range. Positive feedback is not often used in the body, but it is used in blood clotting, sneezing, and generating nerve signals. Another example of positive feedback is uterine contractions during childbirth, as illustrated in Figure 18.11. The hormone oxytocin, made by the endocrine system, stimulates the contraction of the uterus. This pushes the baby’s head toward the cervix, stretching it. The stretched cervix sends a signal to the pituitary gland in the brain to release more oxytocin. The increased oxytocin causes stronger uterine contractions, which push the baby further into the cervix, stretching it more. Increased release of oxytocin, stronger uterine contractions, and further stretching of the cervix continues until the baby is delivered and the positive feedback loop is turned off because the cervix is not longer being stretched as much.
Media Attributions
- Collagen
- Hemoglobin
- Muscle fiber
- Biceps
- Internal Anatomy of the Heart
- Coral reef