18 Background: Mitosis and Meiosis
16.3 Mitosis and Cytokinesis
Mitosis
Karyokinesis, also known as mitosis, is divided into a series of phases—prophase, prometaphase, metaphase, anaphase, and telophase—that result in the division of the cell nucleus.
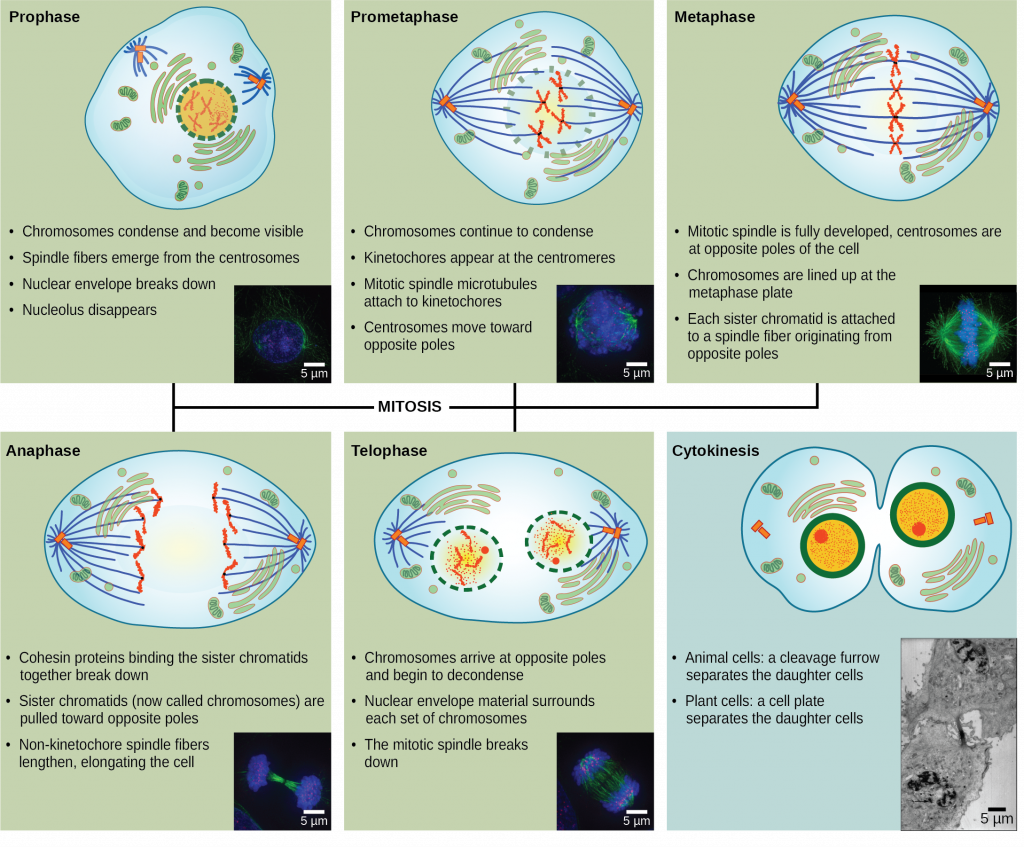
Prophase
Prophase: the nuclear envelope starts to dissociate into small vesicles, and the membranous organelles (such as the Golgi apparatus and the endoplasmic reticulum), fragment and disperse toward the periphery of the cell. The nucleolus disappears as well, and the centrosomes begin to move to opposite poles of the cell. Microtubules that will form the mitotic spindle extend between the centrosomes, pushing them farther apart as the microtubule fibers lengthen. The sister chromatids begin to coil more tightly and now become visible under a light microscope.
Prometaphase
Prometaphase: Many processes that began in prophase continue to advance. The remnants of the nuclear envelope fragment further, and the mitotic spindle continues to develop as more microtubules assemble and stretch across the length of the former nuclear area. Chromosomes become even more condensed and discrete. Each sister chromatid develops a protein structure called a kinetochore in its centromeric region. The proteins of the kinetochore attract and bind to the mitotic spindle microtubules. As the spindle microtubules extend from the centrosomes, some of these microtubules come into contact with and firmly bind to the kinetochores. Once a mitotic fiber attaches to a chromosome, the chromosome will be oriented until the kinetochores of sister chromatids face the opposite poles. Eventually, all the sister chromatids will be attached via their kinetochores to microtubules from opposing poles. Spindle microtubules that do not engage the chromosomes are called polar microtubules. These microtubules overlap each other midway between the two poles and contribute to cell elongation.
Metaphase
Metaphase: All the chromosomes are aligned in a plane called the metaphase plate, or the equatorial plane, roughly midway between the two poles of the cell. The sister chromatids are still tightly attached to each other by cohesin proteins. At this time, the chromosomes are maximally condensed.
Anaphase
Anaphase: The cohesin proteins degrade, and the sister chromatids separate at the centromere. Each chromatid, now called a single chromosome, is pulled rapidly toward the centrosome to which its microtubule is attached. The cell becomes visibly elongated (oval shaped) as the polar microtubules slide against each other at the metaphase plate where they overlap.
Telophase
Telophase: the chromosomes reach the opposite poles and begin to decondense, relaxing once again into a stretched-out chromatin configuration. The mitotic spindles are depolymerized into tubulin monomers that will be used to assemble cytoskeletal components for each daughter cell. Nuclear envelopes form around the chromosomes, and nucleosomes appear within the nuclear area.
Cytokinesis
Cytokinesis, or “cell motion,” is sometimes viewed as the second main stage of the mitotic phase, during which cell division is completed via the physical separation of the cytoplasmic components into two daughter cells However, as we have seen earlier, cytokinesis can also be viewed as a separate phase, which may or may not take place following mitosis. If cytokinesis does take place, cell division is not complete until the cell components have been apportioned and completely separated into the two daughter cells. Although the stages of mitosis are similar for most eukaryotes, the process of cytokinesis is quite different for eukaryotes that have cell walls, such as plant cells, compared to those that don’t.
In animal cells, cytokinesis typically starts during late anaphase. A contractile ring composed of actin filaments forms just inside the plasma membrane at the former metaphase plate. The actin filaments pull the equator of the cell inward, forming a fissure. This fissure is called the cleavage furrow. The furrow deepens as the actin ring contracts, and eventually the membrane is cleaved in two.
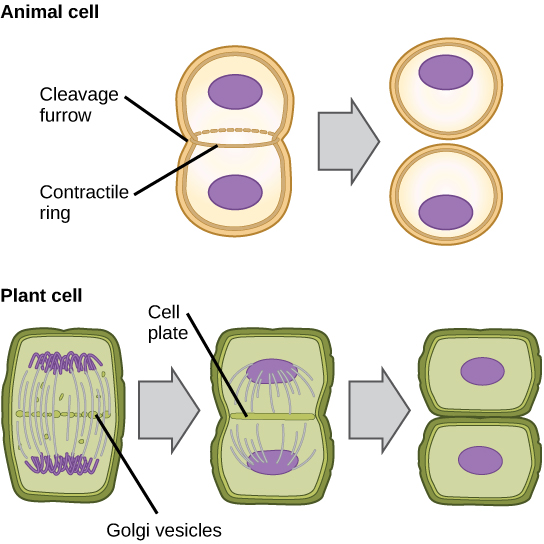
In plant cells, a new cell wall must form between the daughter cells. During interphase, the Golgi apparatus accumulates enzymes, structural proteins, and glucose molecules prior to breaking into vesicles and dispersing throughout the dividing cell. During telophase, these Golgi vesicles are transported on microtubules to form a phragmoplast (a vesicular structure) at the metaphase plate. There, the vesicles fuse and coalesce from the center toward the cell walls; this structure is called a cell plate. As more vesicles fuse, the cell plate enlarges until it merges with the cell walls at the periphery of the cell. Enzymes use the glucose that has accumulated between the membrane layers to build a new cell wall. The Golgi membranes become parts of the plasma membrane on either side of the new cell wall.
18.3 Meiosis
Overview
Meiosis is the nuclear division that forms haploid cells from diploid cells, and it employs many of the same cellular mechanisms as mitosis. However, as you have learned, mitosis produces daughter cells whose nuclei are genetically identical to the original parent nucleus. In mitosis, both the parent and the daughter nuclei are at the same “ploidy level”—diploid in the case of most multicellular animals. In meiosis, the daughter nuclei that result are half of the ploidy level of the parent cell. Most normally, the parent cell is diploid and the daughter cells are haploid.
To achieve this reduction in chromosome number, meiosis consists of one round of chromosome replication followed by two rounds of nuclear division. Because the events that occur during each of the division stages are analogous to the events of mitosis, the same stage names are assigned. However, because there are two rounds of division, the major process and the stages are designated with a “I” or a “II.” Thus, meiosis I is the first round of meiotic division and consists of prophase I, prometaphase I, and so on. Likewise, meiosis II (during which the second round of meiotic division takes place) includes prophase II, prometaphase II, and so on.
Meiosis and fertilization produce unique combinations of genetic material by three mechanisms — genetic recombination during prophase I, independent assortment during metaphase I, and random fertilization.
Meiosis I
Meiosis I separates homologous chromosomes into different daughter cells. The ploidy level of the daughter cells is half of the parent cell; for diploid organisms, the products of meiosis I are haploid.
Interphase
Meiosis is preceded by an interphase consisting of G1, S, and G2 phases, which are nearly identical to the phases preceding mitosis. The G1 phase (the “first gap phase”) is focused on cell growth. During the S phase—the second phase of interphase—the cell copies or replicates the DNA of the chromosomes. Finally, in the G2 phase (the “second gap phase”) the cell undergoes the final preparations for meiosis.
During DNA duplication in the S phase, each chromosome is replicated to produce two identical sister chromatids that are held together at the centromere and are hence part of the same chromosome until anaphase II when they separate from one another.
Prophase I and Crossing Over
Early in prophase I, before the chromosomes can be seen clearly with a microscope, the homologous chromosomes are attached at their tips to the nuclear envelope by proteins. As the nuclear envelope begins to break down, the proteins associated with homologous chromosomes bring the pair closer together. Recall that in mitosis, homologous chromosomes do not pair together. The tight pairing of the homologous chromosomes is called synapsis. In synapsis, the genes on the chromatids of the homologous chromosomes are aligned precisely with each other, which allows the exchange of chromosomal segments between homologous nonsister chromatids—a process called crossing over. Crossing over can be observed visually after the exchange as chiasmata (singular = chiasma).
The crossover events are the first source of genetic variation in the nuclei produced by meiosis. A single crossover event between homologous nonsister chromatids leads to a reciprocal exchange of equivalent DNA between a maternal chromosome and a paternal chromosome. When a recombinant sister chromatid is moved into a gamete cell it will carry some DNA from one parent and some DNA from the other parent. The recombinant chromatid has a combination of maternal and paternal genes that did not exist before the crossover. Crossover events can occur almost anywhere along the length of the synapsed chromosomes. Different cells undergoing meiosis will therefore produce different recombinant chromatids, with varying combinations of maternal and paternal genes. Multiple crossovers in an arm of the chromosome have the same effect, exchanging segments of DNA to produce genetically recombined chromosomes.
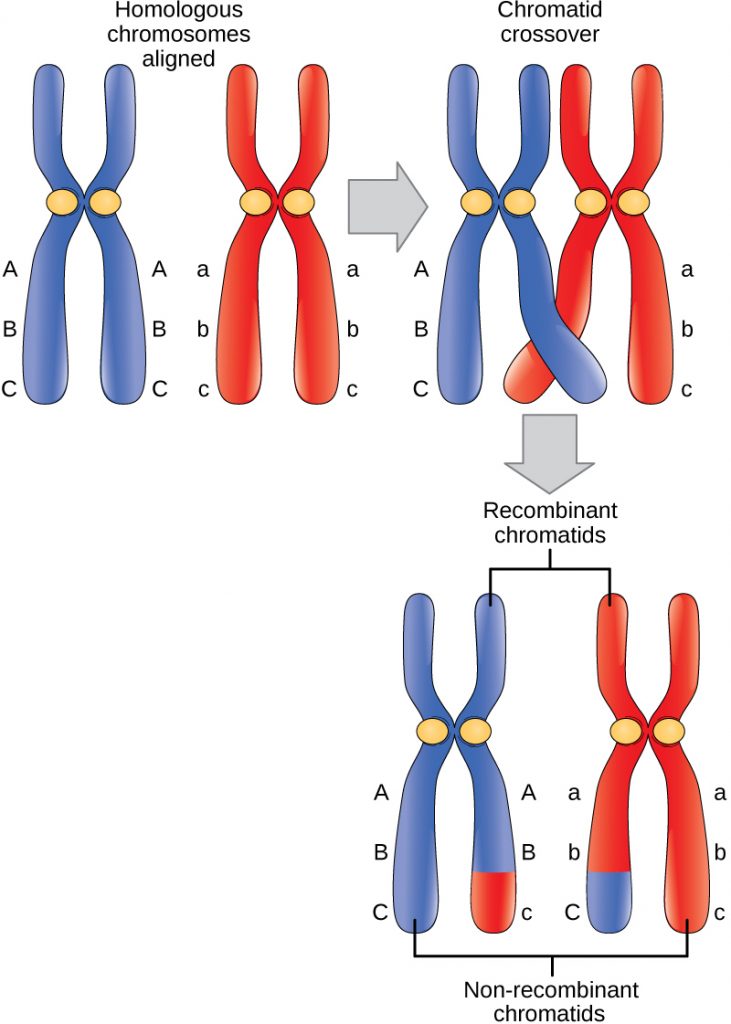
Prometaphase I
The key event in prometaphase I is the attachment of the spindle fiber microtubules to the kinetochore proteins at the centromeres. The microtubules from each pole move toward the middle of the cell and attach to one of the kinetochores of the two fused homologous chromosomes. Each member of the homologous pair attaches to a microtubule extending from opposite poles of the cell so that in the next phase, the microtubules can pull the homologous pair apart.
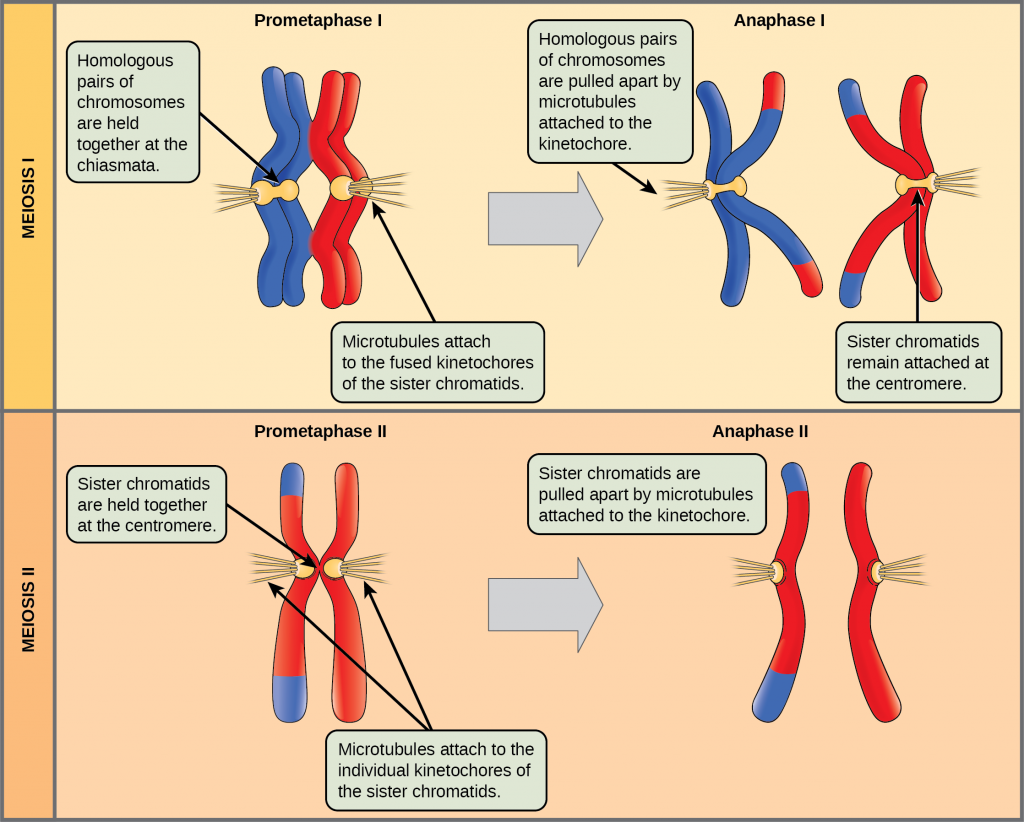
Metaphase I
During metaphase I, the pairs of homologous chromosomes are arranged at the metaphase plate—roughly in the midline of the cell, with the kinetochores facing opposite poles. The homologous pairs orient themselves randomly at the equator. For example, if the two homologous members of chromosome 1 are labeled a and b, then the chromosomes could line up a-b or b-a. This is important in determining the genes carried by a gamete, as each will only receive one chromosome of each homologous chromosome pair. (Recall that after crossing over takes place, homologous chromosomes are not identical. They contain slight differences in their genetic information, causing each gamete to have a unique genetic makeup.)
The randomness in the alignment of recombined chromosomes at the metaphase plate, coupled with the crossing over events between nonsister chromatids, are responsible for much of the genetic variation in the offspring. To clarify this further, remember that the homologous chromosomes of a sexually reproducing organism are originally inherited as two separate sets, one from each parent. Using humans as an example, one set of 23 chromosomes is present in the egg donated by the mother. The father provides the other set of 23 chromosomes in the sperm that fertilizes the egg. Every cell of the multicellular offspring has copies of the original two sets of homologous chromosomes. In prophase I of meiosis, the homologous chromosomes form the tetrads. In metaphase I, these pairs line up at the midway point between the two poles of the cell to form the metaphase plate. Because there is an equal chance that a microtubule fiber will encounter a maternally or paternally inherited chromosome, the arrangement of the tetrads at the metaphase plate is random. Thus, any maternally inherited chromosome may face either pole. Likewise, any paternally inherited chromosome may also face either pole. The orientation of each tetrad is independent of the orientation of the other 22 tetrads.
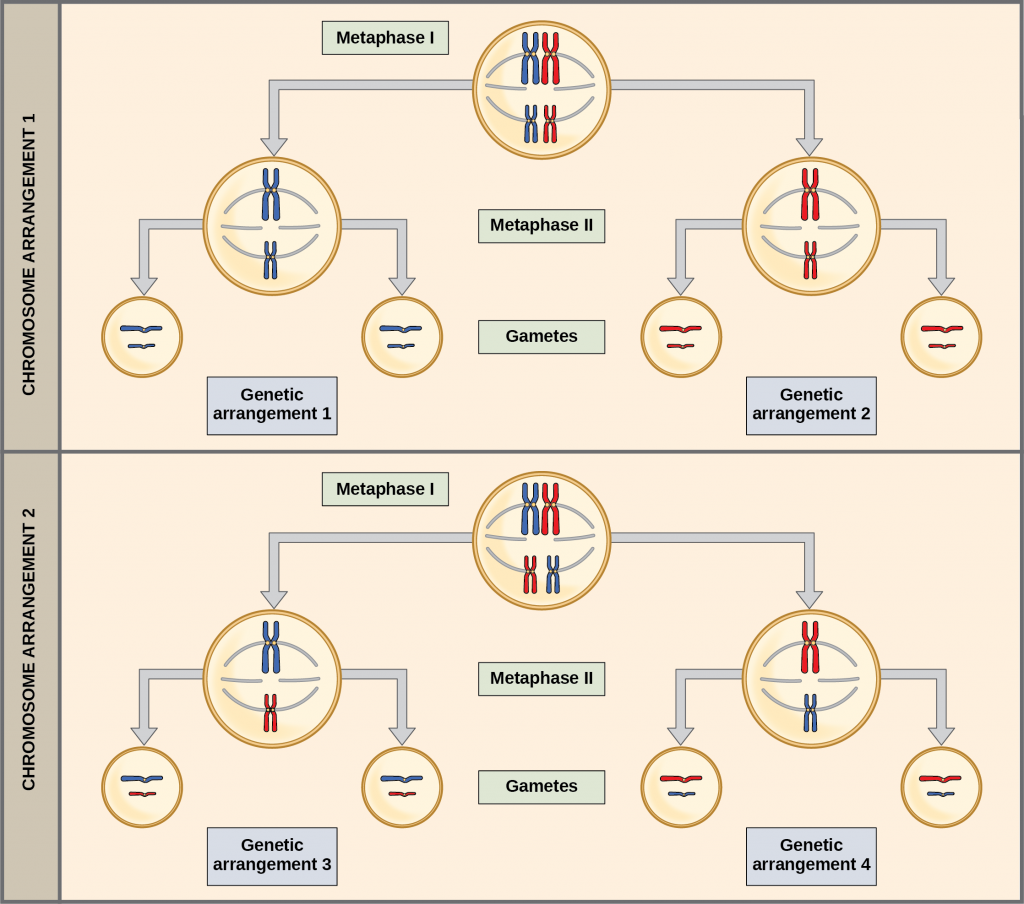
This event—the independent assortment of homologous chromosomes at the metaphase plate—is the second mechanism that introduces variation into the gametes or spores. In each cell that undergoes meiosis, the arrangement of the tetrads is different. The number of variations is dependent on the number of chromosomes making up a set. There are two possibilities for orientation at the metaphase plate; the possible number of alignments therefore equals 2n in a diploid cell, where n is the number of chromosomes per haploid set. Humans have 23 chromosome pairs, which results in over eight million (223) possible genetically-distinct gametes just from the random alignment of chromosomes at the metaphase plate. This number does not include the variability that was previously described (produced by crossing over between the nonsister chromatids). Given these two mechanisms, it is nearly impossible that any two haploid cells resulting from meiosis will have the same genetic composition.
Anaphase I
In anaphase I, the microtubules pull the linked chromosomes apart. The sister chromatids remain tightly bound together at the centromere. The chiasmata are broken in anaphase I as the microtubules attached to the fused kinetochores pull the homologous chromosomes apart.
Telophase I and Cytokinesis
In telophase, the separated chromosomes arrive at opposite poles. The remainder of the typical telophase events may or may not occur, depending on the species. In some organisms, the chromosomes “decondense” and nuclear envelopes form around the separated sets of chromatids produced during telophase I. In other organisms, cytokinesis—the physical separation of the cytoplasmic components into two daughter cells—occurs without reformation of the nuclei.
Two haploid cells are the result of the first meiotic division of a diploid cell. The cells are haploid because at each pole, there is just one of each pair of the homologous chromosomes. Therefore, only one full set of the chromosomes is present. This is why the cells are considered haploid—there is only one chromosome set, even though each chromosome still consists of two sister chromatids. Recall that sister chromatids are merely duplicates of one of the two homologous chromosomes (except for changes that occurred during crossing over). In meiosis II, these two sister chromatids will separate, creating four haploid daughter cells.
Meiosis II
In some species, cells enter a brief interphase, or interkinesis, before entering meiosis II. Interkinesis lacks an S phase, so chromosomes are not duplicated. During meiosis II, the sister chromatids within the two daughter cells separate, forming four new haploid gametes. The mechanics of meiosis II are similar to mitosis, except that each dividing cell has only one set of homologous chromosomes, each with two chromatids. Therefore, each cell has half the number of sister chromatids to separate out as a diploid cell undergoing mitosis. In terms of chromosomal content, cells at the start of meiosis II are similar to haploid cells in G2, preparing to undergo mitosis.
The stages of meiosis II — prophase II, prometaphase II, metaphase II, anaphase II, and telophase II — are similar to the equivalent stages of mitosis.
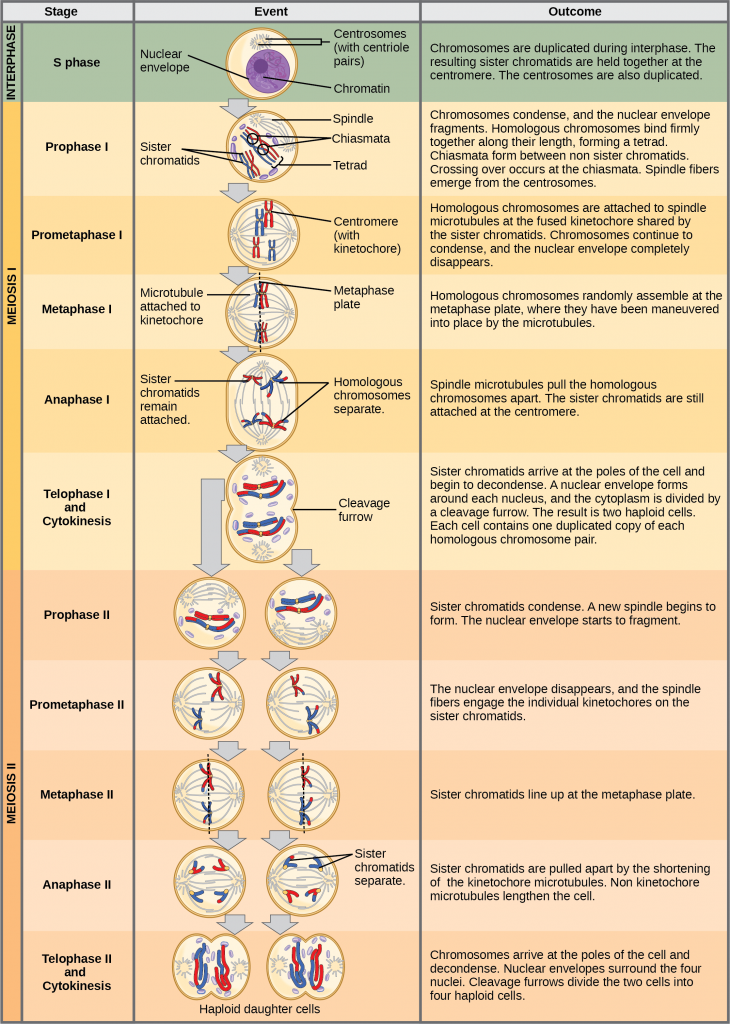
Comparing Meiosis and Mitosis
Mitosis and meiosis are both forms of division of the nucleus in eukaryotic cells. They share some similarities, but also exhibit a number of important and distinct differences that lead to very different outcomes. Mitosis is a single nuclear division that results in two nuclei that are usually partitioned into two new cells. The nuclei resulting from a mitotic division are genetically identical to the original nucleus. They have the same number of sets of chromosomes: one set in the case of haploid cells and two sets in the case of diploid cells. In contrast, meiosis consists of two nuclear divisions resulting in four nuclei that are usually partitioned into four new, genetically distinct cells. The four nuclei produced during meiosis are not genetically identical, and they contain one chromosome set only. This is half the number of chromosome sets in the original cell, which was diploid.
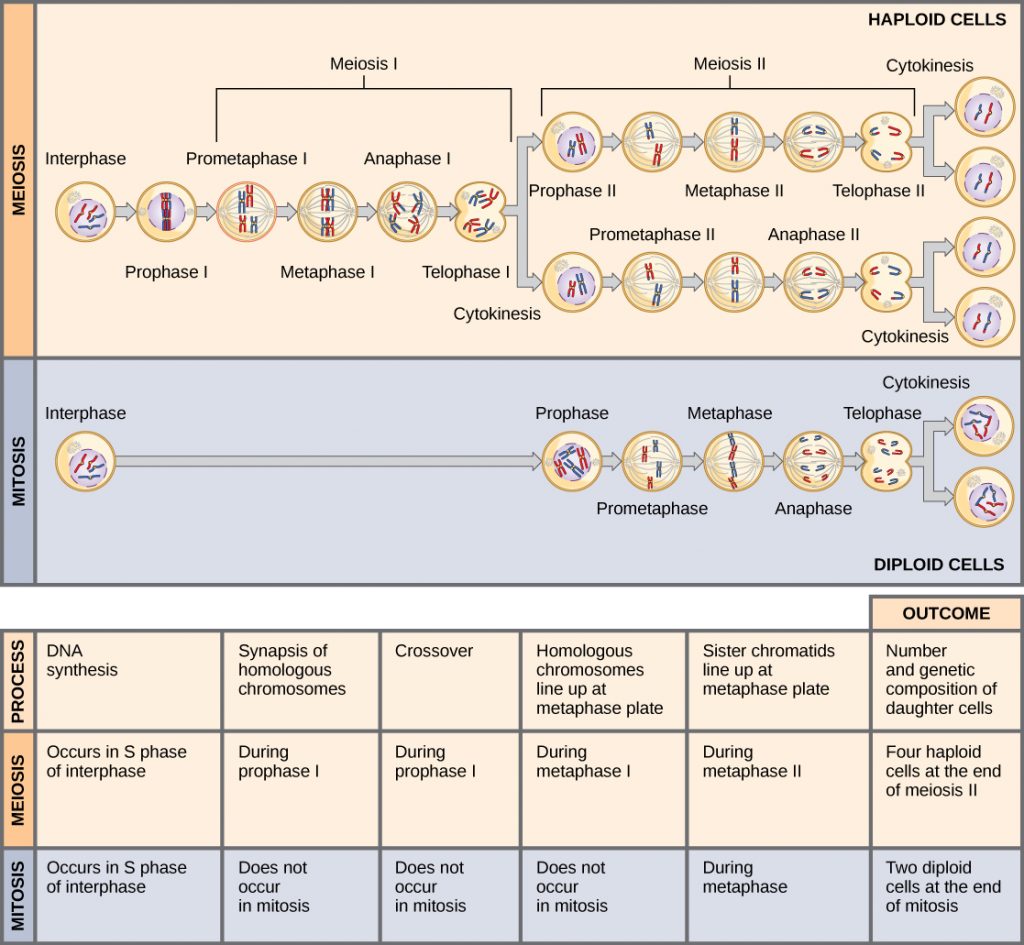